
[PDF]Intermolecular forces Noncovalent interactions

__ Molecular Composition. __ Organic ... Noncovalent interactions. • chemical bonds: ... Relative strengths of intermolecular forces can be seen experimentally:.
Noncovalent Intermolecular Interactions in Organic Electronic Materials: Implications for the Molecular Packing vs Electronic Properties of Acenes
† School of Chemistry and Biochemistry and Center for Organic Photonics and Electronics, Georgia Institute of Technology, Atlanta, Georgia 30332-0400, United States
‡ Department of Chemistry & Center for Applied Energy Research, University of Kentucky, Lexington, Kentucky 40506-0055, United States
§ Solar and Photovoltaics Engineering Research Center, Division of Physical Science and Engineering, King Abdullah University of Science and Technology, Thuwal 23955-6900, Kingdom of Saudi Arabia
Chem. Mater., 2016, 28 (1), pp 3–16
DOI: 10.1021/acs.chemmater.5b03266
Publication Date (Web): October 30, 2015
Copyright © 2015 American Chemical Society
*(C.R.) E-mail: chad.risko@uky.edu., *(J.L.B.) E-mail: jean-luc.bredas@kaust.edu.sa.
Abstract

Noncovalent intermolecular interactions, which can be tuned through the toolbox of synthetic chemistry, determine not only the molecular packing but also the resulting electronic, optical, and mechanical properties of materials derived from π-conjugated molecules, oligomers, and polymers. Here, we provide an overview of the theoretical underpinnings of noncovalent intermolecular interactions and briefly discuss the computational chemistry approaches used to understand the magnitude of these interactions. These methodologies are then exploited to illustrate how noncovalent intermolecular interactions impact important electronic properties—such as the electronic coupling between adjacent molecules, a key parameter for charge-carrier transport—through a comparison between the prototype organic semiconductor pentacene with a series of N-substituted heteropentacenes. Incorporating an understanding of these interactions into the design of organic semiconductors can assist in developing novel materials systems from this fascinating molecular class.
Section 2.3: Non-covalent interactions
Until now we have been focusing on understanding the covalent bonds that hold individual molecules together. We turn next to a brief review on the subject of non-covalent interactions between molecules, or between different functional groups within a single molecule.
2.3A: Dipoles
To understand the nature of noncovalent interactions, we first must return to covalent bonds and delve into the subject of dipoles. Many of the covalent bonds that we saw in section 1.5 – between two carbons, for example, or between a carbon and a hydrogen –involve the approximately equal sharing of electrons between the two atoms in the bond. This is because, in these examples, the two atoms have approximately the same electronegativity. Recall from general chemistry that electronegativity refers to "the power of an atom in a molecule to attract electrons to itself" (this is the definition offered by Linus Pauling, the eminent 20th-century American chemist who was primarily responsible for developing many of the bonding concepts that we have been learning).
Quite often, however, we deal in organic chemistry with covalent bonds between two atoms with very different negativities, and in these cases the sharing of electrons is not equal: the more electronegative atom pulls the two electrons closer to itself. In the carbon-oxygen bond of an alcohol, for example, the two electrons in the s bond are closer to the oxygen than they are to the carbon, because oxygen is significantly more electronegative than carbon. The same is true for the oxygen-hydrogen bond, as hydrogen is slightly less electronegative than carbon, and much less electronegative than oxygen.
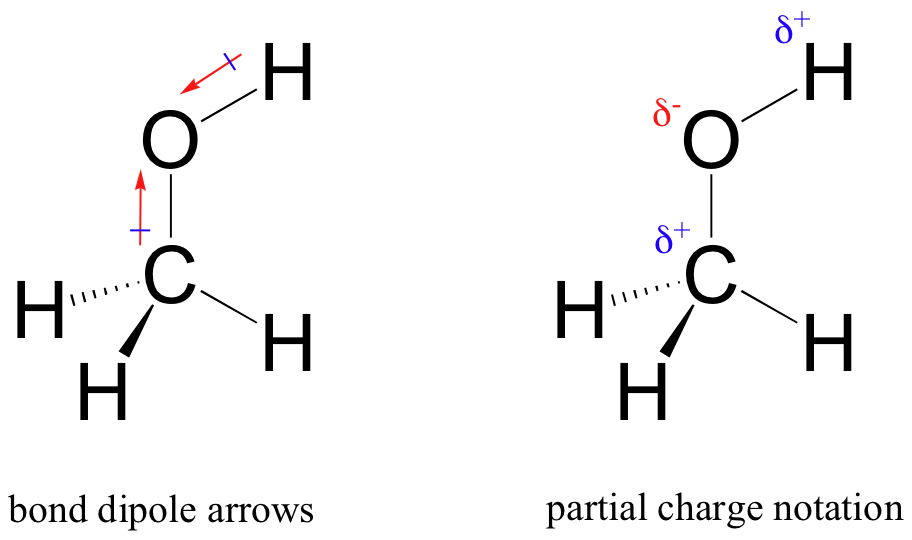
The result of this unequal sharing is what we call a bond dipole, or a polar covalent bond. A polar covalent bond has both negative and positive ends, or poles, where electron density is lower (the positive pole) and higher (the negative pole). The difference in electron density can be expressed using the Greek letter ∂ (delta) to express ‘partial positive’ and ‘partial negative’ charge on the atoms. ‘Dipole arrows’, with a positive sign on the tail, are also used to indicated the direction of higher electron density.
The degree of polarity depends on the difference in electronegativity between the two atoms in the bond. Electronegativity is a periodic trend: it increases going from left to right across a row of the periodic table of the elements, and also increases as we move up a column. Therefore, oxygen is more electronegative than nitrogen, which is in turn more electronegative than carbon. Oxygen is also more electronegative than sulfur. Fluorine, in the top right corner of the periodic table, is the most electronegative of the elements, a property which we will see in later chapters can be exploited by researchers who are trying to work out the details of how organic reactions occur.
Example 2.9
Using the ideas that you have learned about atomic orbitals, rationalize the periodic trends in electronegativity.
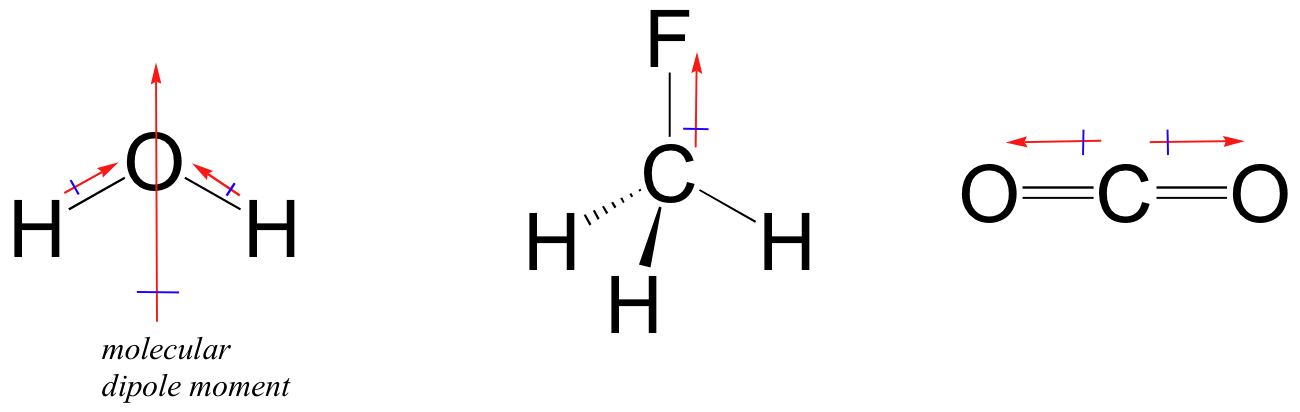
Carbon dioxide, however, has polar bonds that pull in opposite directions, meaning that there is no overall molecular dipole moment.
2.3B: Ion-ion, dipole-dipole and ion-dipole interactions
Arguably the simplest kind of non-covalent interaction is one that we have already discussed: the ionic bond formed by the electrostatic attraction between positively and negatively charged species. One of the most common types of ion-ion interaction in biological chemistry is that between a magnesium ion (Mg+2) and a carboxylate or phosphate group. 2-phosphoglycerate, an intermediate in the breakdown of glucose, provides a good example in the way that it interacts with two Mg+2 ions while binding to an enzyme called enolase.
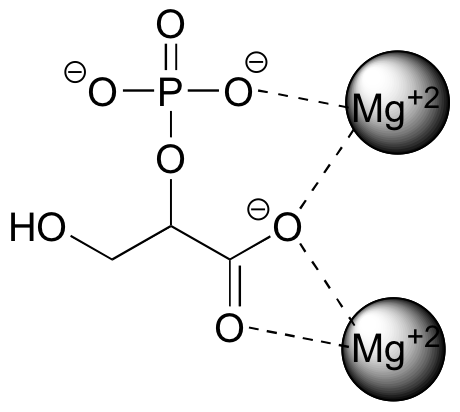
Polar molecules – those with an overall dipole moment, such as acetone – will align themselves in such a way as to allow their respective positive and negative poles to interact with each other. This is called a ‘dipole-dipole interaction.
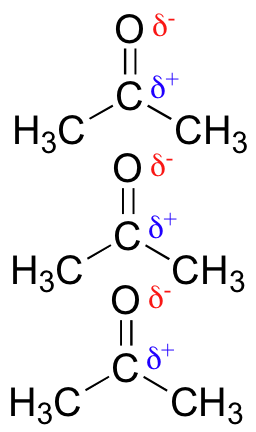
When a charged species (an ion) interacts favorably with a polar molecule or functional group, the result is called an ion-dipole interaction. A common example of ion-dipole interaction in biological organic chemistry is that between a metal cation, most often Mg+2 or Zn+2, and the partially negative oxygen of a carbonyl.

Because the metal cation is very electronegative, this interaction has the effect of pulling electron density in the carbonyl double bond even further toward the oxygen side, increasing the partial positive charge on carbon. As we shall later, this has important implications in terms of the reactivity of carbonyl groups.
2.3C: London dispersion forces
Nonpolar molecules such as hydrocarbons also are subject to relatively weak but still significant attractive intermolecular forces. London dispersion forces result from the constantly shifting electron density in any molecule. Even a nonpolar molecule will, at any given moment, have a weak, short-lived dipole. This transient dipole will induce a neighboring nonpolar molecule to develop a corresponding transient dipole of its own, with the end result that a weak dipole-dipole force is formed. London dispersion forces are relatively weak, but are constantly forming and dissipating among closely-packed nonpolar molecules, and when added up the cumulative effect can become significant. It is through London dispersion interactions that the nonpolar hydrocarbon regions of fat molecules hold together to form the lipid bilayer in cells and subcellular organelles (we will discuss this topic in more detail in section 2.4B)
2.3D: Hydrogen bonds
Hydrogen bonds are an extremely important form of noncovalent interaction. Hydrogen bonds result from the interaction between a hydrogen that is bound to a electronegative heteroatom – specifically a nitrogen or oxygen, or sometimes a fluorine – and a lone pair on a second nitrogen or oxygen. Because a hydrogen is just a single proton with no inner shell (non-valence) electrons, when it loses electron density in a polar bond it essentially becomes an approximation of a ‘naked’ proton, capable of forming a strong interaction with a lone pair on a neighboring oxygen or nitrogen atom.
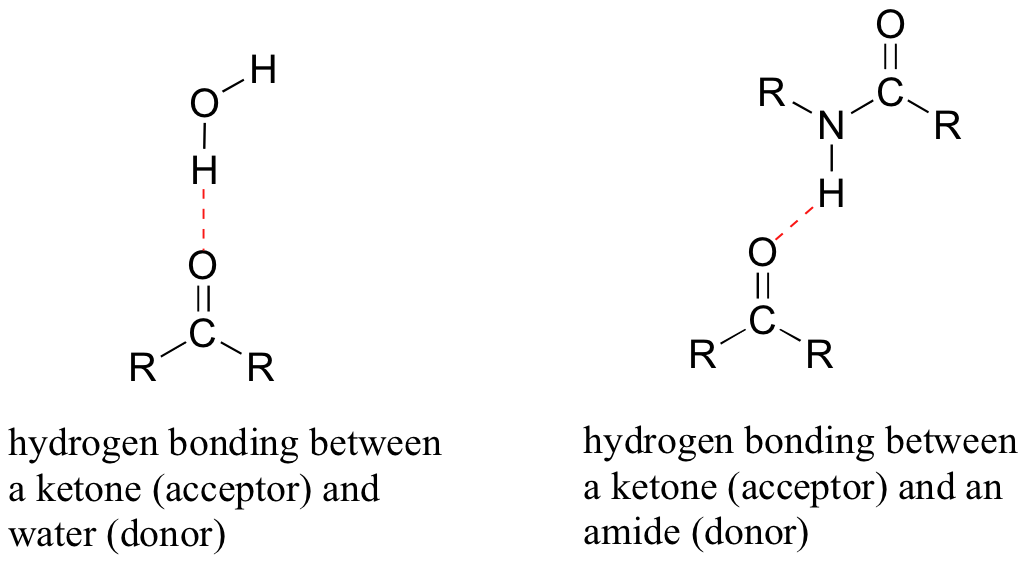
Hydrogen bonds are usually depicted with dotted lines in chemical structures. A group that provides a proton to a hydrogen bond is said to be acting as a hydrogen bond donor. A group that provides an oxygen or nitrogen lone pair is said to be acting as a hydrogen bond acceptor. Many common organic functional groups can participate in the formation of hydrogen bonds, either as donors, acceptor, or both. A water molecule, for example, can be both a hydrogen bond donor and acceptor. A carbonyl, as it lacks a hydrogen bound to an oxygen or nitrogen, can only act as a hydrogen bond acceptor.
Example 2.11
Draw figures that show the hydrogen bonds described below.
- A hydrogen bond between methanol (donor) and water (acceptor).
- A hydrogen bond between methanol (acceptor) and water (donor).
- Two possible hydrogen bonds between methyl acetate and methylamine.
Hydrogen bonds are the strongest type of noncovalent interaction, quite a bit stronger than a dipole-dipole interaction, but also much weaker than a covalent bond. The strength of hydrogen bonds has enormous implications in biology. Genetic inheritance, for example, is based on a very specific hydrogen bonding arrangement between DNA bases: A ‘base-pairs’ with T, while G ‘base-pairs’ with C.
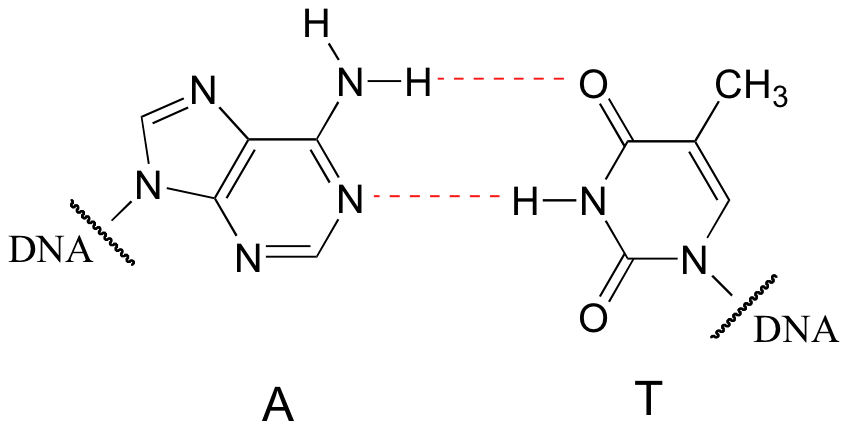
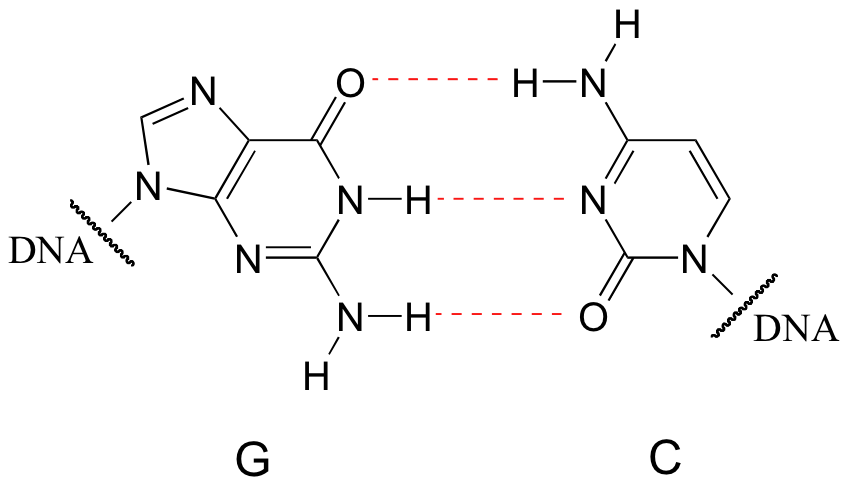
Internal Links
Contributors
- Organic Chemistry With a Biological Emphasis by Tim Soderberg (University of Minnesota, Morris)
No comments:
Post a Comment