http://www.pitt.edu/~jdnorton/teaching/HPS_0410/chapters_2013_Jan_1/origins_pathway/index.html
"...I should observe such a beam of light as an electromagnetic field at rest though spatially oscillating. There seems to be no such thing, however,..."
Chasing a Beam of Light:
Einstein's Most Famous Thought Experiment
John D. Norton
Department of History and Philosophy of Science
University of Pittsburgh, Pittsburgh PA 15260
Homepage: www.pitt.edu/~jdnorton
This page (with animated figures) is available at www.pitt.edu/~jdnorton/goodies
Department of History and Philosophy of Science
University of Pittsburgh, Pittsburgh PA 15260
Homepage: www.pitt.edu/~jdnorton
This page (with animated figures) is available at www.pitt.edu/~jdnorton/goodies
Einstein recalled how, at the age of 16, he imagined chasing after a beam of light and that the thought experiment had played a memorable role in his development of special relativity. Famous as it is, it has proven difficult to understand just how the thought experiment delivers its results. It fails to generate serious problems for an ether based electrodynamics. I propose a new way to read it that fits it nicely into the stages of Einstein's discovery of special relativity. It shows the untenability of an "emission" theory of light, an approach to electrodynamic theory that Einstein considered seriously and rejected prior to his breakthrough of 1905.
For more details, see:
"Chasing the Light: Einstein's Most Famous Thought Experiment," prepared for Thought Experiments in Philosophy, Science and the Arts, eds., James Robert Brown, Mélanie Frappier and Letitia Meynell, Routledge. Download.
Sections 5-6 of "Einstein's Investigations of Galilean Covariant Electrodynamics prior to 1905," Archive for History of Exact Sciences, 59 (2004), pp. 45105. Download.
"Chasing the Light: Einstein's Most Famous Thought Experiment," prepared for Thought Experiments in Philosophy, Science and the Arts, eds., James Robert Brown, Mélanie Frappier and Letitia Meynell, Routledge. Download.
Sections 5-6 of "Einstein's Investigations of Galilean Covariant Electrodynamics prior to 1905," Archive for History of Exact Sciences, 59 (2004), pp. 45105. Download.
1. The Puzzle
How could we be anything but charmed by the delightful story Einstein tells in his Autobiographical Notes of a striking thought he had at the age of 16? While recounting the efforts that led to the special theory of relativity, he recalled
"...a paradox upon which I had already hit at the age of sixteen: If I pursue a beam of light with the velocity c (velocity of light in a vacuum), I should observe such a beam of light as an electromagnetic field at rest though spatially oscillating. There seems to be no such thing, however, neither on the basis of experience nor according to Maxwell's equations. From the very beginning it appeared to me intuitively clear that, judged from the standpoint of such an observer, everything would have to happen according to the same laws as for an observer who, relative to the earth, was at rest. For how should the first observer know or be able to determine, that he is in a state of fast uniform motion? One sees in this paradox the germ of the special relativity theory is already contained."
The thought is simplicity itself. Here is light, a waveform propagating at c:

If the young Einstein were to chase after it at c, he would catch up with the wave and be moving with it, like a surfer riding the wave. He would see a frozen lightwave.
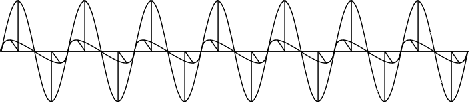
The untenability of that thought led to the downfall of the great achievement of nineteenth century physics, the ether, which then provided the basis for all electromagnetic theory.
The trouble is that it is quite unclear just how this thought creates difficulties for the ether. Einstein gave three reasons and each of them could be answered readily by an able ether theorist.
Einstein wrote... | The ether theorist replies... | |
"...I should observe such a beam of light as an electromagnetic field at rest though spatially oscillating. There seems to be no such thing, however,..." | ||
1 | "...neither on the basis of experience..." | ...but we don't experience frozen light for the simple reason that we are not moving at c through the ether. If we were moving that fast, we would experience frozen light. |
2 | "...nor according to Maxwell's equations..." | Not so. A very short calculation shows that Maxwell's equations predict that light becomes frozen for observers moving at c through the ether. |
3 | "...From the very beginning it appeared to me intuitively clear that, judged from the standpoint of such an observer, everything would have to happen according to the same laws as for an observer who, relative to the earth, was at rest. For how should the first observer know or be able to determine, that he is in a state of fast uniform motion?..." | An observer knows he is moving rapidly with respect to the ether simply because light has become frozen. Analogously a surfer knows he is moving since he stays on the wave. |
So what are we to make of the thought experiment? Perhaps it is no more than the recording of the visceral hunches of a precocious 16 year old who did not even study Maxwell's theory until two years later. This is a possibility we cannot rule out. If it is correct, then we need not puzzle any further over how the thought experiment works, for there is little more to be found that illuminates Einstein's pathway to special relativity.
But then we must ask why the thought experiment merits pride of place in Einstein's defining autobiography? Does it have a cogency that extends beyond Einstein's final high school year? That Einstein mentions Maxwell's equations in the thought experiment suggests their relevance to the operation of the thought experiment and thus that this operation was pertinent to Einstein's later thought, after he had learned Maxwell's equations.
While we cannot know on the evidence available if the thought experiment truly had cogency beyond the mullings of Einstein's 16th year, we can ask if there are plausible accounts of Einstein's pathway to special relativity in which the thought experiment figures more significantly.
2. A Proposed Solution
There is a way of understanding how the thought experiment could have a significance that extended well beyond the confines of Einstein's final year at high school. The key is not to relate the thought experiment to ether theories of electromagnetism. Rather, we know that Einstein devoted some effort during the years leading up to his discovery of 1905, to so-called "emission" theories of light and electromagnetism. Einstein eventually found such theories objectionable and untenable.
I propose that Einstein's thought experiment provided an especially cogent way of formulating those objections and thereby supported Einstein in his final decision: it give up an emission theory in favor of retaining the celebrated Maxwell-Lorentz theory, but with a radically altered theory of space and time.
3. An Emission Theory of Light and Electromagnetism
On many later occasions, Einstein recalled that, prior to his discovery of special relativity, he had investigated emission theories, indicating a similarity in his approach to that used by Walter Ritz. In the then standard electrodynamics of Maxwell and Lorentz, electromagnetic action always propagated at c with respect to the ether. The simplest example was the propagation of a lightwave. But it held equally for the action of one charge upon another. It was this fact that made it seem impossible to conform the principle of relativity to electromagnetism. The ether supplied a preferred state of rest essential to the theory, but incompatible with the idea that all inertial states of motion are equivalent.
So Ritz in 1908, and Einstein sometime before 1905, tried to modify electromagnetic theory in such a way that electromagnetic effects are always propagated at c with respect to the source of the effect. If such a theory could be found, it would no longer require an ether state of rest and it would reasonable to expect that it could conform to the principle of relativity.
The animation below displays the difference. On the left, in the Maxwell-Lorentz theory, electromagnetic action propagates from a fixed point in the ether. So when two charges moving together act on each other, the source of the effect felt by one is a fixed point in the ether left behind by the moving source. Since the effect propagates from a point left behind by the moving charges, an observer moving with the charges can use this fact to determine that the charges are moving.
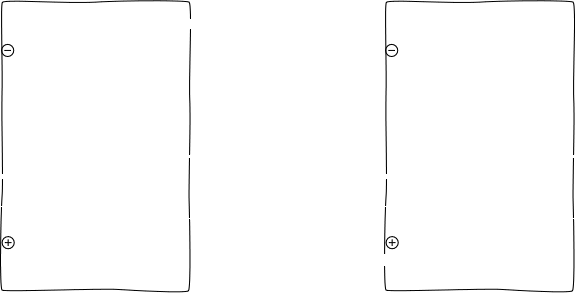
On the right, we see the corresponding process in a modified "emission" theory, such as devised by Ritz and Einstein. The motion of the source is added to the propagating effect. So now the effect propagates isotropically from a point that moves with the source. To see this, notice how the expanding spherical shells remain centered on the moving positive charge that is their source, just as would happen if the two charges were at rest. The propagation of electromagnetic effects can no longer be used by observers moving with the two charges to detect their absolute motion; the principle of relativity is no longer threatened.
The simplest electromagnetic action is the propagation of light. So in this theory, the velocity of the emitter--the source--is added to the velocity of the light emitted. For this reason it is known as an "emission" theory.
Promising as this must initially have seemed to an Einstein intent on restoring the principle of relativity, the emission theory was ultimately rejected by Einstein. His later correspondence and papers are littered with remarks on the problems the theory faced. Two will return as our story unfolds.
- In a letter to Paul Ehrenfest of June 1912 (and elsewhere), Einstein remarked that an emission theory ran afoul of an elementary result of optics: the physical state of a ray of light is determined completed by its intensity and color (and polarization).
- In an interview with R. S. Shankland in the 1950s, Einstein remarked that the theory could not be formulated as a local field theory that is, in terms of differential equations.
- In an interview with R. S. Shankland in the 1950s, Einstein remarked that the theory could not be formulated as a local field theory that is, in terms of differential equations.
In a local field theory, we reconstruct how a field evolves over time by taking its state at one instant and consulting the theory's differential field equations. These equations take the present state of the fields and tell us how rapidly they are changing. From these rates of change we can then infer the states of the field at future times. (A similar analysis tells us how the field will alter at different parts of space.)
4. Einstein's Thought Experiment in the Context of an Emission theory of Light
Let us now return to Einstein's thought experiment and imagine that its target has become an emission theory of light. We immediately see that the three objections Einstein's reports present serious obstacles to an emission theory. Let us take the three objections in order.
1. The first objection was that we don't actually experience frozen light. That is a puzzle in an emission theory of light. We must presume that there are light sources with all sorts of velocities around us. A light source moving rapidly away from us will emit a lightwave that propagates slowly with respect to us. The most extreme case is of light source moving away from us at c. That source will leave a frozen light wave behind in space, as the animation shows:

So, if an emission theory is the correct theory of light, we should expect eventually to run into frozen lightwaves, emitted by rapidly receding sources. But we experience no such thing.
2. The second objection was that frozen light was incompatible with Maxwell's equations. Why should this be a problem for an emission theory when such a theory does not employ Maxwell's equations? It will be a problem, but it takes a few steps to arrive at the conclusion.
First note that an emission theory allows frozen light in ordinary circumstances; we don't need to be moving at c to find it. That means that a frozen light wave must be a part of electrostatics and magnetostatics, the theories of static electric and magnetic fields. Now Maxwell's electrodynamics evolved over the course of half a century and built on a long series of experiments in electricity and magnetism. An emission theory must adjust the theory, but it cannot alter it too radically on pain of incompatibility with those experiments. The one part of Maxwell's theory that seems most secure is its simplest part, its treatment of static electric and magnetic fields. So we would expect a successful emission theory to agree with Maxwell's theory in this simplest and most secure part.
First note that an emission theory allows frozen light in ordinary circumstances; we don't need to be moving at c to find it. That means that a frozen light wave must be a part of electrostatics and magnetostatics, the theories of static electric and magnetic fields. Now Maxwell's electrodynamics evolved over the course of half a century and built on a long series of experiments in electricity and magnetism. An emission theory must adjust the theory, but it cannot alter it too radically on pain of incompatibility with those experiments. The one part of Maxwell's theory that seems most secure is its simplest part, its treatment of static electric and magnetic fields. So we would expect a successful emission theory to agree with Maxwell's theory in this simplest and most secure part.
Now we have a problem: An emission theory allows the existence of frozen light waves. But the emission theory must agree closely with the treatment of static fields in Maxwell's theory and Maxwell's theory does not admit the static fields that corresponds to frozen light waves.
3. In his third objection, Einstein lamented for the observer catching the light beam, "...For how should the first observer know or be able to determine, that he is in a state of fast uniform motion?" Of course, in the context of an emission theory, the "state of fast uniform motion" must be read as "fast uniform motion with respect to the source of the light."
At first it is not clear why it should matter at all whether the observer catching the light beam can make this judgment. It turns out to be important if the overall emission theory of light is to be deterministic; that is, if the present state of fields and the like in space are to be able to determine how they will develop in the future. Einstein's worry is that determinism will fail. To see why, imagine that you are an observer given a waveform, but all you know of it is its state at the present instant.
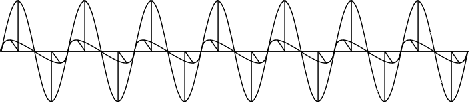
Light wave at an instant
Would you be able to tell whether the waveform is one that is frozen in space;
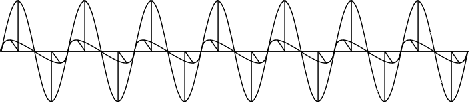
One possible future: a frozen light wave
or whether it is one that is propagating past you?

Another possible future: a propagating light wave
Both are possible in an emission theory. Which is the case depends upon your velocity with respect to the light's source. If you are moving at c with respect to the source, the wave is frozen. If you are at rest with respect to the source, the wave is propagating at c.
Can you tell which case you have by merely looking at the waveform at an instant? You cannot. Einstein's earlier remark about light is now decisive. A light wave is fully characterized by its color, intensity and polarization and both cases agree on these properties. The waveform has no property at an instant that would enable you to tell what its future time development would be. This is indeterminism. The present state of the wave does not determine its future time development.
While this circumstance might just be just an odd incompleteness of our knowledge, it becomes a crisis if we imagine that we are not human observers but the differential equations of a local field theory. For, as we saw above, a basic function of those field equations is to take the present state of the fields and from them infer the rates of change of the field. Those rates of change then determine the time development of the waveform--whether it propagates or not and how fast it propagates. This essential function will not be possible in an emission theory, for the instantaneous state of the lightwave does not determine the rates of change of the field.
Hence, thanks to Einstein's thought experiment, we infer that an emission theory cannot be formulated as a local field theory.
We can summarize the problems brought by Einstein's thought experiment to an emission theory:
Einstein wrote... | The emission theorist worries... | |
"...I should observe such a beam of light as an electromagnetic field at rest though spatially oscillating. There seems to be no such thing, however,..." | ||
1 | "...neither on the basis of experience..." | An emission theory allows frozen waveforms for observers in all inertial states of motion, so we should expect to experience them. |
2 | "...nor according to Maxwell's equations..." | An emission theory should agree closely on static fields with Maxwell's theory, but Maxwell's theory prohibits the static fields of frozen light (except in the special case of observers moving at c with respect to the ether). |
3 | "...From the very beginning it appeared to me intuitively clear that, judged from the standpoint of such an observer, everything would have to happen according to the same laws as for an observer who, relative to the earth, was at rest. For how should the first observer know or be able to determine, that he is in a state of fast uniform motion?..." | We cannot tell from the instantaneous state of a light wave whether it is a frozen wave or a propagating wave. So differential field equations cannot tell either and and an emission theory of light cannot be formulated as a local field theory governed by differential field equations. |
5. Conclusion
When Einstein abandoned an emission theory of light, he had also to abandon the hope that electrodynamics could be made to conform to the principle of relativity by the normal sorts of modifications to electrodynamic theory that occupied the theorists of the second half of the 19th century. Instead Einstein knew he must resort to extraordinary measures. He was willing to seek realization of his goal in a re-examination of our basic notions of space and time. Einstein concluded his report on his youthful thought experiment:
"One sees that in this paradox the germ of the special relativity theory is already contained. Today everyone knows, of course, that all attempts to clarify this paradox satisfactorily were condemned to failure as long as the axiom of the absolute character of time, or of simultaneity, was rooted unrecognized in the unconscious. To recognize clearly this axiom and its arbitrary character already implies the essentials of the solution of the problem."
Copyright John D. Norton, December 2004. Rev. February 15, 2005. Reformatted April 14, 2005 on a transatlantic flight returning to Pittsburgh from the Israel Academy of Science and Humanities conference on Einstein.
HPS 0410 | Einstein for Everyone |
Back to main course page
Einstein's Pathway to Special Relativity
Background reading: J. Schwartz and M. McGuinness, Einstein for Beginners. New York: Pantheon.. pp. 1 - 82.
We have now reviewed the developments in the physics of moving bodies, of light, of electricity and magnetism that brought the physics that Einstein found when he began to think about ether, electricity, magnetism and motion.
It was pondering these developments that led Einstein to discover the special theory of relativity in 1905. The discovery was not momentary. The theory was the outcome of, in Einstein's own reckoning, seven and more years of work. He even places one of his early landmarks in a thought experiment he had at the age of 16, in 1896, nine years before the year of miracles of 1905. Unfortunately we have only fragmentary sources to document the years of this struggle. Below I identify a few of the major ones.
The story of Einstein's discovery of special relativity has exercised an almost irresistible fascination on many, in spite of the dearth of sources. So, if you read more widely, you will see much speculation over how to fill in the blanks between the known landmarks and even over which are the important landmarks. Some of it is responsible; some is not.
Chasing a beam of light

Einstein in high school
Writing a half century later in 1946 in his Autobiographical Notes, Einstein recounted a thought experiment conducted while he was a 16 year old student in 1896 that marked his first steps towards special relativity.
"...a paradox upon which I had already hit at the age of sixteen:
If I pursue a beam of light with the velocity c (velocity of light in a vacuum), I should observe such a beam of light as an electromagnetic field at rest though spatially oscillating.
There seems to be no such thing, however, neither on the basis of experience nor according to Maxwell's equations.
From the very beginning it appeared to me intuitively clear that, judged from the standpoint of such an observer, everything would have to happen according to the same laws as for an observer who, relative to the earth, was at rest. For how should the first observer know or be able to determine, that he is in a state of fast uniform motion?
One sees in this paradox the germ of the special relativity theory is already contained."
If I pursue a beam of light with the velocity c (velocity of light in a vacuum), I should observe such a beam of light as an electromagnetic field at rest though spatially oscillating.
There seems to be no such thing, however, neither on the basis of experience nor according to Maxwell's equations.
From the very beginning it appeared to me intuitively clear that, judged from the standpoint of such an observer, everything would have to happen according to the same laws as for an observer who, relative to the earth, was at rest. For how should the first observer know or be able to determine, that he is in a state of fast uniform motion?
One sees in this paradox the germ of the special relativity theory is already contained."
The basic thought is clear. If Einstein were to chase after a propagating beam of light at c

he would see a frozen light wave

and that Einstein deemed impossible.
At first it seems that is will be simple to figure out just what is worrying Einstein. He states a few simple reasons. I don't want to go into them here since they actually turn out to be rather hard to disentangle. My best effort to disentangle them is given at "Chasing a Beam of Light: Einstein's Most Famous Thought Experiment," http://www.pitt.edu/~jdnorton/Goodies/Chasing_the_light
Magnet and conductor
Einstein's thinking evolved from this early, youthful flight into richer and technically more detailed scrutiny of motion in Maxwell's electrodynamics. Einstein initially took the idea of an ether state of rest seriously and conceived experiments that were designed to reveal the earth's motion through the ether.
These thoughts eventually took a very different turn with Einstein deciding that the ether state of rest had no place in electrodynamics and that the principle of relativity was to be upheld. The decisive moment seems to have come with a thought experiment, the magnet and conductor, that is recounted in the opening paragraph of Einstein's 1905 paper.
This is a version of that thought experiment that is modified slightly from the way Einstein sets it up. (Caution!) | The simple idea behind the thought experiment is that Maxwell's electrodynamics treats a magnet at rest in the ether very differently from one that moves in the ether. A magnet at rest is surrounded by a magnetic field only. |

However, if | through the ether, things are very different. In addition to the magnetic field, a new entity comes into being around the magnet, an induced electric field.The creation of the electric field draws on details of Maxwell's theory that need not distract us here. Briefly, as the magnet moves past a fixed point in the ether, the magnetic field strength changes with time at that point. That change in field strength, according to Maxwell's theory, creates an electric field. |

This difference between the two cases seems to provide an unequivocal marker of motion through the ether--or so it would seem. To determine if a magnet is moving absolutely through the ether or not, one merely needs to look for that induced electric field. That is easy to do. An electric field accelerates electric charges, such as the conducting electrons in a piece of wire, a conductor. So all that has to be done is to place a conductor near the magnet, as the figures show, and to look for an induced electric current. If there is one, then there is an induced electric field and magnet is moving; if there isn't one, then the magnet is at rest in the ether.
It all seems so straightforward. But it doesn't work. The simplest situation arises if we attach the conductor to the magnet so that it moves or rests with the magnet. If the magnet is at rest in the ether, then there will be no current in the conductor. So far, it is as expected. But if the magnet and conductor move together an extra complication enters. Because the conductor is now moving absolutely in a magnetic field, another part of Maxwell's theory tells us that a second electric current will be induced in the conductor. Remarkably that second current flows in the opposite direction to the one produced by the electric field and it turns out to cancel it out exactly.
The upshot is that checking for an electric current in the conductor fails as a means of distinguishing the absolute rest of the magnet from its motion. In both cases, the current is the same--no current at all. So an Einstein riding with an absolutely moving magnet, would detect no current and find the situation to be indistinguishable from absolute rest as far as the observable currents were concerned.

More curiously, it is as if the electric field just isn't there for an observer moving with the magnet. But one at rest in the ether would say there is an electric field present.

Einstein later described how this realization had affected him quite profoundly:
"In setting up the special theory of relativity, the following ... idea concerning Faraday’s magnet-electric induction [experiment] played a guiding role for me... [magnet conductor thought experiment described]. ...The idea, however, that these were two, in principle different cases was unbearable for me. The difference between the two, I was convinced, could only be a difference in choice of viewpoint and not a real difference. Judged from the [moving] magnet, there was certainly no electric field present. Judged from the [ether state of rest], there certainly was one present. Thus the existence of the electric field was a relative one, according to the state of motion of the coordinate system used, and only the electric and magnetic field together could be ascribed a kind of objective reality, apart from the state of motion of the observer or the coordinate system. The phenomenon of magneto-electric induction compelled me to postulate the (special) principle of relativity. [Footnote] The difficulty to be overcome lay in the constancy of the velocity of light in a vacuum, which I first believed had to be given up. Only after years of [jahrelang] groping did I notice that the difficulty lay in the arbitrariness of basic kinematical concepts." | Einstein, Albert (1920) “Fundamental Ideas and Methods of the theory of Relativity, Presented in Their Development,” Collected Papers of Albert Einstein, Vol. 7, Doc. 31.![]() Einstein in 1920 |
In sum Einstein's lesson was this. Maxwell's theory employed an ether state of rest; but that state of rest could not be revealed by observation. So somehow the principle of relativity needed to be upheld.
In retrospect, this relativity of the induced electric field had, in effect, committed Einstein to the relativity of simultaneity, although he certainly did not know it at the time. A simple thought experiment shows that it can only be reconciled with Maxwell's electrodynamics if we give up the absoluteness of simultaneity. See From the Magnet and Conductor to the Relativity of Simultaneity on my "Goodies" page. | And a second moral was an unexpected relativity. Prior to Einstein, it had been thought that whether an electric field is present at some place is an absolute fact. Einstein now concluded that it is observer dependent: some observers will judge an electric field to be present; others in a different state of motion will not. This was the first of Einstein's reorganization of our ideas of which quantities are absolute and which relative. |
Emission theories of light
The magnet and conductor thought experiment marked the way forward for Einstein. He was to uphold the principle of relativity in electrodynamics. The only obvious way of doing that was to modify electrodynamical theory. As the concluding footnote in Einstein's quote from 1920 above suggests, Einstein could already know one element that must be in the modification. According to Maxwell's theory, light always propagates at c with respect to the ether. That result must change if the theory conforms to the principle of relativity since there will no longer be an ether state of rest against which the motion of the light can be judged.
![]() Walther Ritz | We know from later recollections what one of Einstein's modified versions of electrodynamics looked like. In that version, the velocity of light is a constant, not with respect to the ether, but with respect to the source that emits the light. Such a theory is called an "emission" theory of light and, if the other parts of the theory are well behaved, will satisfy the principle of relativity. |
Einstein later recalled that the theory he developed was essentially that developed later by Walther Ritz in 1908. In Ritz's theory--and thus probably also in Einstein's theory--all electrodynamic action, not just light, propagated in a vacuum at c with respect to the actions source. The essential change is shown in the animation: | For experts: the way to built the theory was actually very easy. If Maxwell's theory is formulated in terms of retarded potentials, one needs only to tinker with the formula for the retardation time to bring the whole theory into the form of an emission theory. Everything else can stay the same. |
In Maxwell's theory, all electrodynamic action, generated by a source charge at some moment, propagates at c from the fixed point in the ether occupied by the source at that moment.
| ![]() |
In a Ritz-style emission theory, all electrodynamic action, generated by a moving source, propagates at c from a point that moves at uniform velocity with the source.
|
Here is a non-animated version:

My own best effort to reconstruct of the details of Einstein's theory can be found in "Einstein's Investigations of Galilean Covariant Electrodynamics prior to 1905," Archive for History of Exact Sciences, 59 (2004), pp. 45-105.
Crisis: the relativity of simultaneity
It was a lovely theory. But it didn't work. We can only guess what the problems were. But we know he found many. Indeed Einstein seems to have expended considerable energy trying to figure out if any emission theory might work. His later recollections are littered with different reasons for why no emission theory at all could do justice to electrodynamics. | My own conjectures on how these arguments may have worked are discussed in part in my"Chasing a Beam of Light:Einstein's Most Famous Thought Experiment," |
An emission theory fails. So Einstein would have found himself in an impossible position. The speed of light cannot vary with the speed of the emitter; presumably it must be a constant, as Maxwell's theory had urged all along. Yet in addition, Einstein was convinced that the principle of relativity must obtain in electrodynamic theory. How can both obtain? They require the speed of light to be the same for all inertial observers?
The footnote already quoted above points us to Einstein's next step.
"The difficulty to be overcome lay in the constancy of the velocity of light in a vacuum, which I first believed had to be given up. Only after years of [jahrelang] groping did I notice that the difficulty lay in the arbitrariness of basic kinematical concepts."
"The difficulty to be overcome lay in the constancy of the velocity of light in a vacuum, which I first believed had to be given up. Only after years of [jahrelang] groping did I notice that the difficulty lay in the arbitrariness of basic kinematical concepts."
The key to the puzzle is the relativity of simultaneity. If Einstein gives up the absoluteness of simultaneity, then the principle of relativity and the constancy of the speed of light are compatible after all. The price paid for the compatibility is that we must allow that space and time behaves rather differently than Newton told us.
More importantly for Einstein's struggles of that time is an extra bonus: it turns out that within the new theory of space and time of special relativity, Maxwell's electrodynamics does not need to be modified at all. It turns out to be compatible with principle of relativity just as it is. That would have been a very satisfactory outcome for Einstein.
Einstein recounted later the moment of discovery. In a lecture in Kyoto on December 14, 1922, he is reported by Ishiwara, who took notes in Japanese, to have said:
"Why are these two things inconsistent with each other? I felt that I was facing an extremely difficult problem. I suspected that Lorentz’s ideas had to be modified somehow, but spent almost a year on fruitless thoughts. And I felt that was puzzle not to be easily solved. But a friend of mine living in living in Bern (Switzerland) [Michele Besso]helped me by chance. One beautiful day, I visited him and said to him: ‘I presently have a problem that I have been totally unable to solve. Today I have brought this “struggle” with me.’ We then had extensive discussions, and suddenly I realized the solution. The very next day, I visited him again and immediately said to him: ‘Thanks to you, I have completely solved my problem.” My solution actually concerned the concept of time. Namely, time cannot be absolutely defined by itself, and there is an unbreakable connection between time and signal velocity. Using this idea, I could now resolve the great difficulty that I previously felt. After I had this inspiration, it took only five weeks to complete what is now known as the special theory of relativity." Translation from Stachel, John (2002) Einstein from ‘B’ to ‘Z.’: Einstein Studies, Volume 9. Boston: Birkhäuser, p. 185. | ![]() Einstein taking sake ![]() A portrait of Einstein by the cartoonist Okamoto Ippei (1886-1948), done in December of 1922 in Sendai, Miyagi Prefecture, Japan |
![]() David Hume | This moment of recognition of the relativity of simultaneity is one of the great moments of discovery in science and, at this moment philosophical reflections played a key role. Absolute simultaneity seems an uncontroversial part of the world. How could we give it up? Einstein had been reading many philosophers, including Hume and Mach. They had stressed that concepts are our servants, not our masters, and they are warranted only in so far as they might be grounded in experience. So was absolute simultaneity grounded properly in experience? Einstein began to think about the experiences that we use to establish simultaneity of events and he realized that it was not. Reading these philosophers gave him the courage to continue and to abandon absolute simultaneity. In its place came the relativity of simultaneity. | ![]() Ernst Mach |
For an account of how reading Hume and Mach helped, see my "How Hume and Mach Helped Einstein Find Special Relativity."
The turn to principles
The moment of the recognition of the relativity of simultaneity came, in the above account, 5 weeks prior to Einstein's completion of the 1905 paper (and in another 5 to 6 weeks). In these five to six weeks in which he pulled together the pieces of the finished theory, Einstein made one more very significant methodological advance that would forever color how we see relativity theory.
Einstein's pathway to discovery amounted to the recognition that if you take Maxwell's electrodynamics seriously you have to see that built into it is both the principle of relativity and a new kinematics of space and time that supports it. Yet Einstein does not simply argue it that way in the finished paper.
![]() | The reason is not hard to see. Prior to, just a few months before completing his 1905 special relativity paper, Einstein had published a paper in which he had foreshadowed the demise of Maxwell's electrodynamics! In his earlier light quantum, Einstein had advanced the astonishing assertion that sometimes light does not behave like a wave as Maxwell's theory demanded; sometimes it behaved like a spatially localized collection of energy. |
So how could Einstein now base a new theory of space and time on Maxwell's theory? He knew something was very right about Maxwell's theory. There was also something very wrong about it. How could one theorize in such an unstable environment. The answer came to Einstein, as he reported in his Autobiographical Notes, in a distinction of what he called constructive theory from theories of principle.
"Reflections of this type made it clear to me as long ago as shortly after 1900, i.e., shortly after Planck's trailblazing work, that neither mechanics nor electrodynamics could (except in limiting cases) claim exact validity. Gradually I despaired of the possibility of discovering the true laws by means of constructive efforts based on known facts. The longer and the more desperately I tried, the more I came to the conviction that only the discovery of a universal formal principle could lead us to assured results. The example I saw before me was thermodynamics. The general principle was there given in the theorem: The laws of nature are such that it is impossible to construct a perpetuum mobile (of the first and second kind). How, then, could such a universal principle be found?"
In effect, what Einstein saw was that he did not really need all of Maxwell's theory for his new account of space and time. He needed only a few core ideas robust enough to survive the coming quantum revolution. Following the model of thermodynamics, these few core ideas would be advanced as principles from which the entire theory could be deduced.
What could those principles be? The principle of relativity itself was an obvious choice. He also needed something that distilled the relevant essence of Maxwell's electrodynamics. What about the hardest won lesson of his years of work towards the final theory: the recognition that an emission theory of light must fail? That is, that Maxwell's theory was right after all in demanding that that light always propagates at c, no matter how fast the emitter may be moving? That became the second principle, light postulate. Those two principles proved to be sufficient to allow the entire theory to be deduced. Einstein laid out both as his postulates and the theory adopted its now familiar form.
Three Components
We have seen three components in Einstein's discovery:
- Astute analysis of new and surprising experiments.
- Deeply reflective philosophical analysis of the nature of time and physical theories.
- Solving an incongruous and overlooked problem in the foundations of electricity and magnetism.
While all three had a role in Einstein's discovery, the last was the most decisive. Unfortunately this is often overlooked in accounts of the origins of Einstein's theory. Einstein's engagement with current experiments and his facility in philosophical analysis are important. However special relativity would not have come about at all were it not for the particular problems in electrodynamics addressed by Einstein and which demanded a radical solution.
Einstein's 1905 "On the Electrodynamics of Moving Bodies"

Einstein arrived at his "On the electrodynamics of moving bodies," which is my best candidate for the most famous scientific paper ever written. | An online version of this paper is here. Beware of a famous misrendering in this standard edition as noted in this version of the first two sections. |
The paper has several parts. First there is an introduction. It commences with the recounting of the magnet and conductor thought experiment. It then announces the project of solving the resulting problem with a new theory of space and time based on the principle of relativity and the light postulate.
In the first "Kinematical Part" of the paper, Einstein develops the parts of the theory devoted only to space and time. Its first section, "Definition of Simultaneity," Einstein gives his celebrated analysis of the relativity of simultaneity. It is one of the most celebrated conceptual analyses of the century and a model very many others tried to follow. | ![]() |
![]() | The second "Electrodynamical Part" proceeds to what must have seemed for Einstein in 1905 to be the real benefit of the paper. He proceeded to show how Maxwell's electrodynamics was already a theory that conformed to the principle of relativity and noted that this fact made solution of some problems in electrodynamics very easy. For a problem concerning moving systems, such as the reflection of light off a moving mirror, was really the same as another much easier problem with resting bodies, such as the reflection of light off a resting mirror. If you could solve the easy problem, then the principle of relativity let you write down a solution to the harder one almost immediately, just by transforming your viewpoint from one frame of reference to another. |
For more on Einstein's discoveries of 1905, see my website.
What you should know
- What Einstein at age 16 imagined it would be like to chase light.
- His magnet and conductor thought experiment and what he learned from it.
- How he tried to use emission theories of light.
- The importance of his insight on simultaneity.
- Why he chose to formulate the special theory in terms of two principles.
Copyright John D. Norton. January 2001, September 2002; July 2006; January 2, 2007; January 21,February 4, 2008; January 15, 2010.
No comments:
Post a Comment