量子態隱形傳輸 並把每個光子之兩個自由度,動量和極化態,一齊考慮進來,他們在一個光子上應用這兩個自由度建構了不同的貝爾態,而要傳輸的極化態 是在其中一個光子上調出來的,他們成功地把極化態 由一個光子傳輸到另一個光子上,這兩光子的傳輸裝置只能傳輸純態,非純態就無能為力了
双光子复合量子态的隐形传输
量子态的隐形传输借助于粒子对的纠缠,它在量子通信和量子计算中有重要应用. 相互纠缠的粒子之间关联极强, 无论两粒子相隔多么遥远, 它们的状态只能用二者的连立行为描述, 而不可能被单独描述. 中国科大郭光灿教授曾用身处异国的母女来比喻纠缠关联: 身在美国的女儿生下了自己的孩子, 生活在中国的母亲, 其身份自然地变更为母亲兼外婆. 也就是说, 女儿的变化必然决定母亲的变化. 1997年以来, 单光子极化态的隐形传输已经分别在实验室内和户外(长距离)被实现. 所谓”隐形”是指, 在量子态从一个粒子( x光子) 传给另一个粒子( b光子) 的过程中, 不必事先知道x光子的态. 信息的发送者Alice与 信息的接收者Bob共同拥有纠缠光子对( a和b ), Alice要把自己一边的x光子的极化态传给Bob一方的 b光子. Alice首先对 a光子和 c光子进行所谓 “Bell 态联立测量”, 由于a和b纠缠, 这一测量使b光子自动获得了关于x光子极化态的信息. 然后, Alice把测量结果通过经典通道(如, 长途电话)告诉Bob, 而Bob只需据此对b光子做一次变换, 便可使b光子具有与x光子初始态全同的量子态. 此时, x光子初始态已因 “Bell 态联立测量” 而被破坏, 因此上述传态不违背”量子态不可克隆” 的限制.
最近, 中国科大潘建伟小组在隐形传态领域又取得了突破性进展. 他们首次实现了双光子(x1和 x2)复合量子态的隐形传输, 并且x1和 x2的初始态可以相互关联. 在实验中Alice拥有的(a1和 a2)与Bob拥有的(b1和b2)形成两个纠缠对, 起并行传输通道的作用. 最终, (x1和 x2)复合量子态传给了(b1和b2). 实验者巧妙地解决了一个个技术难题, 其中包括同时操控六光子纠缠态.
量子隱形傳輸
文/李建二
摘 要
近十年來「量子資訊(quantum information)」開始萌芽發展,本文針對其中重要之一環「量子隱形傳輸」(quantum teleportation) 作入門之介紹。
|
一、引言
很多人看過「星際航行(star trek)」這部電視連續劇。我第一次觀賞這個節目是在三十年前正在美國求學的時候,看到「航海家(Voyager)」星艦上的艦長柯克(Kirk)站在「傳輸室(transporter)」內,室外一位艦員按下控制鍵,柯克便罩在耀眼的光芒下,很快變淡,成稀疏光點而消逝,隨即在星艦下星球之某處(預先選定),先出現光點,再模糊形象,然後柯克出現了,這情境相信在很多人心理留下深刻的印象和懂憬,整個過程包含實体分解、實体傳輸及實体組合等三個步驟,人類的科學是否真的能夠建構這樣的設備?也許, 在遙遠(?)的未來,近十年來「量子資訊(quantum information)」開始萌芽發展,物理學家已經可以初步做到把一個光子的狀態傳輸到另一個不相干的光子上,這就是本文要介紹的「量子隱形傳輸」[1]。
二、什麼是量子隱形傳輸 ?
量子隱形傳輸是量子計算和量子資訊中重要的一環,它們都嘗試應用量子系統來從事計算、儲存及傳輸資訊,任一量子系統所處的狀態稱之為量子態,在不同的環境和刺激下,可以處於不同的量子態,量子隱形傳輸廣義來說如下:把系統A之任一量子態經過一套設備完整地傳輸到系統B。這不是拷貝,因為原來系統A之量子態在傳輸後完全被破壞了。但這樣的定義也包含了這種情形:系統A和系統B間可以相互作用,如果排除這種可能,就得到一般所認知的量子隱形傳輸:系統A和系統B 可以相距遙遠(譬如在銀河之兩端),彼此間無任何作用之可能。在這樣的情況下,把系統A之任一量子態經過一套設備(包括古典通訊)完整地傳輸到系統B。要大約了解這是如何做到,也就是它的機制,則先要介紹一些量子系統完全異於古典系統的特殊性質。
三、量子態
前面提到的量子系統,它的演變是依據量子理論來進行,其量子態也完全由量子理論來決定。 實際上所有在巨觀世界及微觀世界的系統都是量子理論適用的範疇,只是在巨觀世界裡量子的行為一般都不顯著,在這樣的情形下,量子理論趨近於古典理論而運用到巨觀現象。而在微觀世界理,古典理論不能適用,微觀現象只能用量子理論來描述。所以這裡所提的量子系統都是微觀世界裡的系統,如分子、原子、電子、光子、量子點(quantum dot)、輻射場等。以下將以光子為例說明量子態及其特殊性。
從量子理論的觀點,電磁波是由一群光子所組成。每一光子具有動量及兩個極化態(polarization)。這三者互相垂直,我們把這兩個極化態叫做水平極化態和垂直極化態,分別以
及
表示之並以之作為基本態,任意之極化態則是基本態之任意線性疊合如下:
其中
和
是任意複數,
代表在狀態
之機率,且其總機率為壹:
。 亦即當你對此光子做測量時得到水平極化態之機率是
,而得到垂直極化態之機率是
。但在量子理論中,測量(measurement)有其特殊性:如果你量到的是水平極化態,則測量後,此光子就保持在此水平極化態,亦即原本之狀態
在測量時被破壞而崩潰(Collapse)成測量後之水平極化態
。所以一次的測量,是無法決定
,而且又把
破壞了,這就是說如果你想把一個你不知道的光子狀態傳輸給別人,你想靠測量此光子以獲得
及
,進而告知對方進而重組是不可能的。
四、糾纏態
上一節介紹了一個光子的量子態。本節將介紹由兩個光子組成的系統之量子態。我們一樣只考慮光子的極化態,並選擇
為基底(即基態之集合)。其中底標1(2)代表第一(二)個光子, 兩-光子之極化態可以分為兩類,第一類是兩個光子的極化態各自獨立互不關連,其一般式可寫成
, 其中
且
上面的基態都屬於此類。 第二類是兩個光子的極化態不能寫成各自狀態之張量乘積(tensor product),亦即
。 我們稱之為兩-光子之糾纏態(entangled state)。在糾纏態中,兩個光子之極化態互相關連,不受時空之限制,亦即具有非侷域性關連(non-local correlation)。我們也可以選擇四個正規(orthonormal) 糾纏態作為基底,常用的稱之為貝爾態(Bell states),其形式如下:
,
(3)
我們以
糾纏態為例來進一步說明,在此態中它告訴我們第一個光子在水平極化態而第二個光子在垂直極化態之機率是1/2,同樣的第一個光子在垂直極化態而第二個光子在水平極化態之機率也是1/2,因此各別光子之極化態是任意(Random)而未知的,但如果我們對第一個光子進行測量而得到水平極化態,則第二個光子就自動地瞬間地處在垂直極化態,不管它離第一個光子有多遠(譬如在銀河的另一端),這就是所謂的非侷域性關連, 這種現象是完全非古典的,貝爾狀態又稱為 EPR 偶(EPR Pairs),其中E代表愛因斯坦,PR是另外兩位物理學家(B. Podolsky and N. Rosen),他們三人於1935 [2]年發表一篇著名的論文質疑非侷域性關連及量子力學之完整性,引起相當熱烈的論辨,後來實驗肯定了非侷域性關連的存在。
五、貝爾態測量
一個光子的水平或垂直極化態可以用檢極器(Analyzer)或極化分光器(polarized beam splitter)來分析, 如果測量的結果是水平極化, 則測量後此光子原先之狀態
即崩潰而變成水平態
,對兩個光子之系統,我們也可以去量各別光子的水平或垂直極化態,如果測量的結果是第一個光子是水平態,第二個光子是垂直態,則測量後此系統之原先狀態便崩潰成
態,但我們不一定要量光子的水平或垂直極化態,也可以直接設法去測量兩光子之貝爾態,則測量後此兩光子系統便處在所量到的貝爾態上,這就是所謂的貝爾態測量(Bell state measurement)。兩光子貝爾態測量中,主要的設備是 50:50 分光器(beam splitter)。當兩光子同時抵達分光器時,兩光子之波包( wave packet)相互重疊,因而產生干涉( interference)效應,每一個光子經過分光器後可能繼續前進或被反射,調整分光器使兩光子離開分光器後之兩個途徑之對應狀態相互疊合,以用一個分光器作貝爾態測量為例如圖一,
我們在光子離開分光器(BS)出來的兩個途徑上各置一個光子偵測器(detector) d1及d2,並僅對兩個偵測器同時偵測到光子時作記錄,這就是所謂的同時量測(coincidence measurement)。這樣的設置意謂每一出來的途徑必須有一個光子,所以只有兩個可能:兩個光子經過分光器時都繼續前進或同時被反射,因為這兩種情形是無法區分的,所以出來後之兩光子態是這兩種情形之狀態之線性疊合(linear superposition),其振幅(amplitude)大小相同而異號,因為兩光子之任意狀態都可以上述之四個正交(orthogonal)的貝爾態之線性疊合來表示,但觀察這些貝爾態在把兩個光子對調下,只有
變號,其它保持不變,所以在這樣的實驗設置下,只有貝爾態
是容許的,其他都相互抵消了,也就是量到了一個貝爾態
,而原來兩光子之狀態也崩潰成此貝爾態了。
我們在光子離開分光器(BS)出來的兩個途徑上各置一個光子偵測器(detector) d1及d2,並僅對兩個偵測器同時偵測到光子時作記錄,這就是所謂的同時量測(coincidence measurement)。這樣的設置意謂每一出來的途徑必須有一個光子,所以只有兩個可能:兩個光子經過分光器時都繼續前進或同時被反射,因為這兩種情形是無法區分的,所以出來後之兩光子態是這兩種情形之狀態之線性疊合(linear superposition),其振幅(amplitude)大小相同而異號,因為兩光子之任意狀態都可以上述之四個正交(orthogonal)的貝爾態之線性疊合來表示,但觀察這些貝爾態在把兩個光子對調下,只有
六、量子隱形傳輸之機制
於1993年由IBM 特別研究員(IBM Fellow) Charles H. Bennett [3] 領導的國際研究團隊共六人,聯名發表一篇文章,證明在原先系統上之狀態被破壞下,完全的量子隱形傳輸在理論上是實際可能的。在此之前,科學家們並不認為它真的可行,因為它破壞了量子力學中的測不準原理(uncertainty principle)。這個原理說明不可能經由一次測量而得知系統之所有資訊,譬如對一個粒子的位子量的愈正確,則它的動量就愈不正確, 且對該粒子之干擾也愈嚴重,終於完全破壞了該粒子之原先狀態且無法得知所有資訊,因此無法據以再造一個具有完全相同狀態的粒子,所以認為完全的量子隱形傳輸是不可能的,但是Bennett 的研究團隊應用量子力學中所特有的糾纏態,不必要去量知原先粒子之所有資訊而在理論上達成完全的量子隱形傳輸,底下我們描述這個機制。
假定甲、乙、丙三個人各自擁有一個粒子,分別稱為1、2、3粒子,甲扮演委託人的角色,在粒子1上製造了一個狀態
七、量子隱形傳輸之實驗
Bennett 研究團隊所提出的機制一直到1997年才在澳大利亞Innsbruck 大學由Anton Zeilinger [4] 所領導的研究團隊第一次作出來。 Innsbruck 實驗成功地把一個光子的任意極化態完整地傳輸到另一個光子上, 但是成功的機率只有25%,這是因為在作貝爾態測量時,他們用的方法就是上面所介紹的貝爾態測量,這個方法只能量到一個貝爾態
,其他三個貝爾態則量不到,因為四個貝爾態出現的機率是相同的,所以成功地傳輸的機率只有25%,Innsbruck 實驗之基本架構如圖二。其後在1998年初,在義大利羅馬大學的研究團隊[5]應用兩個糾纏的光子,並把每個光子之兩個自由度,動量和極化態,一齊考慮進來,他們在一個光子上應用這兩個自由度建構了不同的貝爾態,而要傳輸的極化態
是在其中一個光子上調出來的,他們成功地把極化態
由一個光子傳輸到另一個光子上,這兩光子的傳輸裝置只能傳輸純態,非純態就無能為力了,同年年底,在美國加州理工學院由Jeff Kimble[6]領導的光學研究團隊發表了一個完全成功的量子隱形傳輸,只是他們用的不是三個或兩個光子而是幅射場(Radiation Field),所用的狀態不是極化態或動量而是一致態(coherent state),在他們的裝置下,可以測量所有的貝爾態,因此甲送進之狀態皆能傳輸到丙,傳輸機率為100%。
八、未來展望
以上實驗所完成的量子隱形傳輸都是在同樣的量子系統間傳輸狀態,而且用的量子系統都是光子(電磁場),未來應可以發展到不同的量子系統,如電子,原子,分子,量子點等,而且不限於同樣量子系統間狀態之傳輸,我們如果能夠把一個不穩定或壽命短的系統上之資訊傳輸到一個穩定且壽命長的系統上,就能夠發展量子記憶(quantum memory),當然我們都希望能像「航海家」星艦上一樣地傳輸人或實物,也許在遙遠的未來會成功,但是實体之傳輸應該完全不同於狀態之傳輸,其困難度是難於估計的,除此以外,量子隱形傳輸也預期將在量子電腦及密碼學方面扮演重要的角色。 走近量子纠缠-18-量子隐形传输(一)

||
无论是量子信息、量子密码、量子计算等等,所有想要在计算或通讯中应用量子力学规律的领域,都离不开一个基本的位元:量子比特。从前面的章节我们已经了解到,量子比特是一个量子态,由于量子态的叠加性质,n个量子比特能够表示的状态数比n个经典比特能表示的状态数多得多,因此,量子比特比经典比特听起来更强大、更有用多了。不过,我们也知道,量子态是不确定的、难以对付的。除此之外,它还有个经典比特完全没有的性质:不可克隆定理。
量子态不可克隆定理说: 一个未知的量子态是不可克隆的。有学者在1982年(见参考资料)从量子态叠加原理的推论,而证明了这个定理。在此,我们只是从测不准原理来粗浅地理解这个定理:从经典‘克隆’的意义上说,要想精确地复制一个物品,首先就要得到(测量)这个物品的所有的信息。然而,对一个遵循量子规律的系统(比如量子比特),我们不可能同时精确测量它的所有物理量,因为根据“海森堡测不准原理”,在同一时刻以相同精度测定量子的位置与动量是不可能的,我们只能精确测定两者之一。
从量子论的观点而言,测不准原理应该被称为“不确定性原理”更恰当一些。但如果使用经典的图像来想象微观世界的话,叫做“测不准”可能还更容易理解。比如,以测量电子为例,所谓测量,一定要使用测量方法和工具,要对电子进行测量,最好的方法就是使用激光去与电子相互作用。原子中的一个电子,从经典角度看,它的运动轨道是如此之小(10-10米),它的运动速度又是如此之快(106米/秒),在这种快速运动情形下的电子,被测量它的光子顶头一撞,速度和位置都全变了,又怎么可能测得准呢?
比如说,利用光被电子散射,可以测量电子的位置,但不可能将粒子的位置确定到比光的波长更小。所以,要想将位置测量准确,必须用更短的波长的光,而波长更短的光子具有更大的能量,就对电子的速度产生更大的扰动,使得速度更不能测准,反过来说也是一样。
“量子不可克隆定理”,是指在不知道量子状态的情况下复制单个量子是不可能的,因为要复制单个量子就只能先作测量,而测量必然改变量子的状态。我们在介绍量子比特时提到过,一个qubit有两个自由度,由于测不准原理的限制,我们无法准确地测量这两个自由度,因此也就无法精确地克隆出这个量子比特的状态。
量子态不可克隆,这是在通讯中使用量子比特的极大优越性。这个优点保证了量子密码、量子通讯的安全性。但是,也由此而为它在通讯上的真正应用设置了难以逾越的障碍。在我们现代社会中铺天盖地的通讯网中,每秒钟都在复制、传输着天文数字个比特的信息。仅拿一台ADSL上网的计算机来说吧,如果网速是512Kbps,那就是每秒钟传输51.2万个比特。可是,量子比特怎么办呢?连复制都不行,如何传输呢?
科学家总能想出一些窍门,不能克隆没关系,我们照样传输它们!这就是近年来在这个行业内热门的话题,叫做“量子隐形传输”。
美国的国际商业机器公司(IBM)不愧是计算机行业的龙头老大,它不仅引领着传统的经典计算机的研发和制造,在量子计算机的研究方面,几十年来也独树一帜,不论在理论方面,还是实验方面,都进行了大量的研究工作。比如上一节中提到过的“可逆计算” 的IBM科学家R. Landauer,他在1961年对“可逆计算”的研究就与量子计算机研究有关。
“量子隐形传输”的理论设想,是由另一位IBM研究中心的研究员,查尔斯·亨利·贝内特最先提出来的。贝内特1943年生于美国纽约市,既是一位物理学家,又是信息理论学家,是现代量子信息理论的开山鼻祖之一。
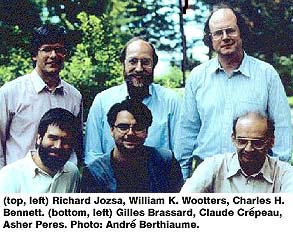
贝内特等提出“量子隐形传输”的六人团队
照片来自网络:http://researcher.ibm.com/view_project.php?id=2862
贝内特1970年从哈佛大学得到博士学位后,于1972年加入IBM的研究队伍。在IBM,他做了大量有关量子信息学方面的工作。他曾经提出对麦克斯韦妖的重新解释,他与同行们合作开发了BB84量子密码协议,并建立了世界上第一个量子密码的工作演示。
1993年,Bennett等六人团队,在“物理评论快讯”上发表文章,提出“量子态隐形传输”的设想。设想将一个未知量子态的完整信息,合作通过两个独立的通道(经典和量子)发送出去,在新的远离的位置重新组合后,产生一个在发送过程中被破坏了的原始量子态的精确副本。
贝内特等人的想法可由下图说明:
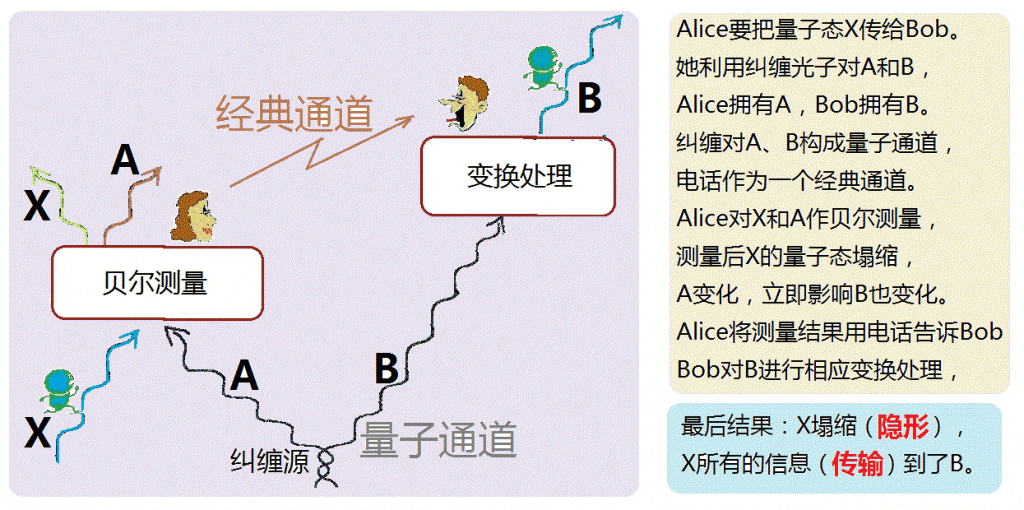
图中左边的Alice,想要把量子态X传给Bob。她利用纠缠光子对A和B,Alice拥有纠缠光子中的A,而Bob拥有B。纠缠光子A、B构成量子通道,电话或是互联网可作为经典通道。首先,Alice对需要传送的X和她手中的A作“贝尔测量”。测量后,X的量子态塌缩了,A也发生变化。因为A和B互相纠缠,A的变化立即影响B也发生变化。然而,Bob无法察觉B的变化,直到从经典通道得到Alice传来的信息。比如说,Alice在电话中将测量结果告诉Bob。然后,Bob对B进行相应的变换处理。最后,B成为和原来的X一模一样。这个传输过程完成之后,X塌缩隐形了,X所有的信息都传输到了B上,因而称之为“隐形传输”。
读者从上面的说法中,可能会提出以下几个问题:
1。既然是仍然要使用经典的通道,那为何还要量子通道呢?用经典通道把全部信息都传过去好了。
2。在Alice这边的方框中,“贝尔测量”是什么意思?
3。在Bob那边的方框中,“变换处理”是什么意思?
提出第一个问题的人,一定是因为不记得“量子不可克隆定理”了!根据这个原理,我们是不可能得到量子态X的全部信息的,所以,从经典通道就不可能传递所有信息。实际上,我们可以用经典电传(FAX)的例子作比喻,就知道,要想得到经典物体的“所有信息”,是很困难的。
用电传机发送电传的过程,可以用上面量子隐形传输示意图简化,而得到如下图所示的“电传示意图”:
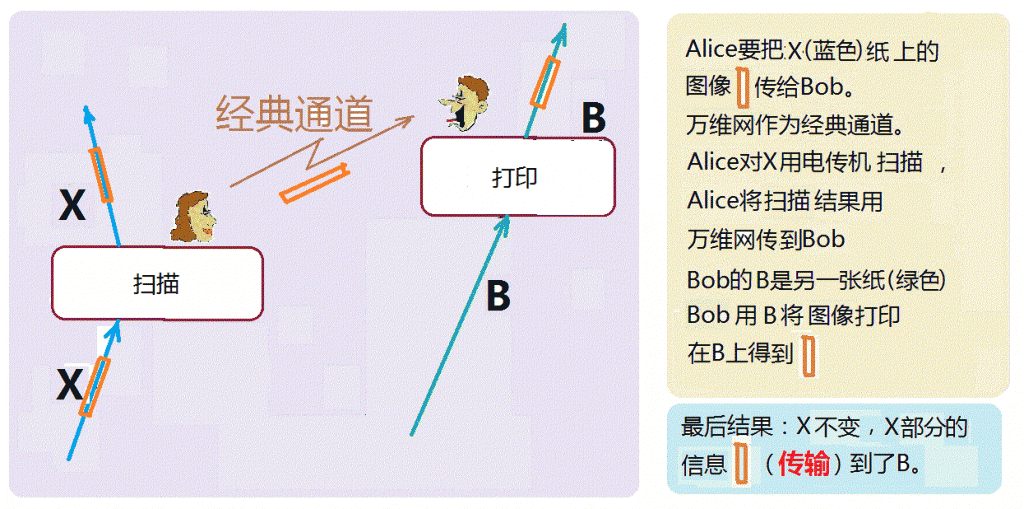
从上图看到,比之“量子隐形传输”,电传过程少了一个纠缠对构成的量子通道。在电传过程中,首先,Alice将上面印有图像信息的蓝色纸X进行扫描,得到需要传输的图像信息。然后,将此信息从经典通道(互联网)传给Bob。Bob收到图像后,用另外一张纸B(绿色)将图像打印出来。在这种传递过程中,“图像”只是X的一部分信息,X的其他信息,诸如纸张材料、颜色、大小、厚度等等,并不能从扫描过程得到,也没有被传递过去。况且,即使是Bob知道了这些性质,造出一张表面看起来完全一样的纸来打印图像,后来的B也不能说是和原来的X一模一样的。因为肯定不可能保证每个分子都一样吧。
而在量子隐形传输中,最后的B是和原来的X完全一样的。换言之,电传时传输后所复制出来的,只是纸上图像的信息,没有复制出任何‘实体’本身。量子隐形传输却有点像是:从得到实体的完整信息,而复制出了‘实体’本身,尽管只是一个小小的量子态!这样说,人们可能要心情激动、欢呼雀跃:“啊!科幻电影中远距离传物的时代就要来临了!” 其实远远不是这样,那种想法是一个误解。我们这儿谈论的“复制”不过只是一个量子现象,完全不知道如何才能复制一个较大的、真正的物体。即使是海边一颗小小的沙粒的传输复制,也还相距十万八千里。
上面提到的与量子通道有关的“贝尔测量”以及“变换处理”,留到下节讲解。
参考资料:
量子不可克隆定理:Wotte rs W . K . and Zurek W . H ., Nature . 299 (1982 ) , 802
量子隐形传输:C.H. Bennett, G. Brassard, C. Crepeau, R. Jozsa, A. Peres, and W. Wootters, "Teleporting an Unknown Quantum State via Dual Classical and EPR Channels", Phys. Rev. Lett. vol. 70, pp 1895-1899 (1993)
http://blog.sciencenet.cn/blog-677221-550786.html 此文来自科学网张天蓉博客,转载请注明出处。
上一篇:走近量子纠缠-17-量子计算机
下一篇:走近量子纠缠(19)量子隐形传输(二)
走近量子纠缠(19)量子隐形传输(二)
精选

||
在解释贝尔测量(Bellmeasurement)之前,首先复习一下介绍qubit时使用过的狄拉克符号,并且重温我们在第八节中提到过的贝尔态的定义。
对一个单光子的系统,考虑它所有的偏振态,可以表示为两个基态|1>和|0>的线性组合:|A> = a|1>+b|0>。如果是两个光子的系统,就有4个基态:
|11>、|10>、|01>、|00>。 (19.1)
这个两光子系统的所有量子态都可以用这4个基底的线性组合来表示。此外,我们也可以采取另外一种基底,叫做贝尔态基底。这就如同在我们的3维空间中,我们可以将xyz坐标轴旋转成另外一套x’y’z’坐标轴一样。这样做的目的是将原来那套不纠缠的基底(19.1)换成4个纠缠态(贝尔态)作为基底。4个贝尔态在原来的(19.1)基底下,可以表示如下面的形式:
|f+> =|11>+|00> (19.2)
|f-> =|11>-|00> (19.3)
|y+> =|10>+|01> (19.4)
|y-> =|10>-|01> (19.5)
既然(19.2-19.5)是2粒子量子态态空间的基底,那么,所有2粒子的量子态就都可以表示成这4个贝尔态的线性组合,也就是这4个贝尔态的叠加态:
|2粒子量子态> = B1|f+> + B2|f-> + B3|y+> + B4|y-> (19.6)
(19.6)中的B1、 B2、 B3、 B4为复数,它们绝对值的平方:|Bn|2,分别表示测量时,这个2粒子量子态塌缩到相应的贝尔态的几率。因此,所谓的Alice对两个光子作“贝尔测量”的意思,就是探测这个两粒子系统到底塌缩到哪一个贝尔态。
在实验室里,用作两光子贝尔态测量的主要设备是50:50分光器(beam splitter)。当一个光子经过分光器后,可能继续前进(透射),也可能被反射。光子走任何一条道路的几率是50%。这种分光器输出的各种情形如下图所示。
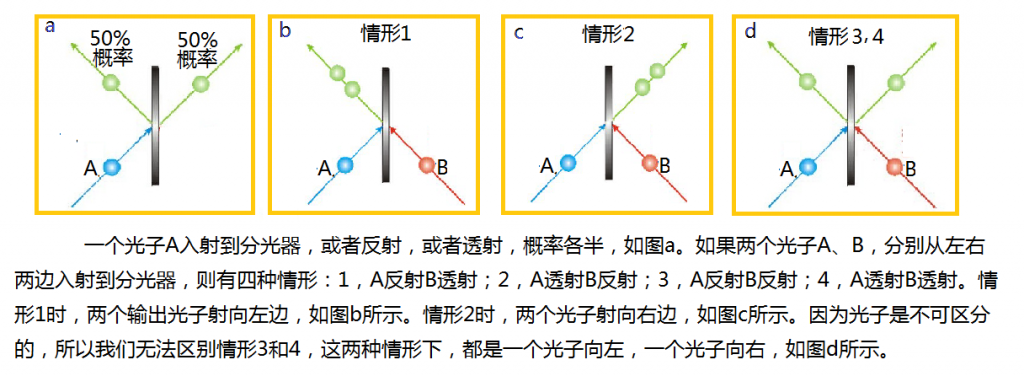
上图中的图a表示:一个光子A入射到分光器,或者反射,或者透射,概率各半。
现在考虑两个光子A和B,分别从左右两边入射到分光器。当两光子同时抵达分光器时,两光子之波包相互重叠,因而产生干涉效应。它们经过分光器后有四种情形:1,A反射、B透射;2,A透射、B反射;3,A反射、B反射;4,A透射、B透射。第一种情形,两个输出光子同时射向左边,如图b所示。情形2时,两个光子同时射向右边,如图c所示。但是,我们无法区别第三和第四这两种情形,因为光子是不可区分的。我们不知道,从分光器射出的光子,哪个来自A?哪个来自B?所以,在3和4这两种情形下,都是一个光子向左,一个光子向右,如图d所示。
在此还必须说明一点:仅仅利用线性光学器件,不可能在实验中区分4个贝尔态。理论上已经证明,最多只可能区别4个贝尔态中的3个。所以,也就是说,如果只用线性元件,我们就只能作“不完全的贝尔测量”。在上面的公式(19.2-19.5)所表示的4个贝尔态中,|y-> =|10>-|01>是一个反对称的单态,另外的|f+>、|f->和|y+>则构成对称的三态。利用刚才所介绍的半透半反分光器,可以将贝尔单态|y->,与其它贝尔态分开来。
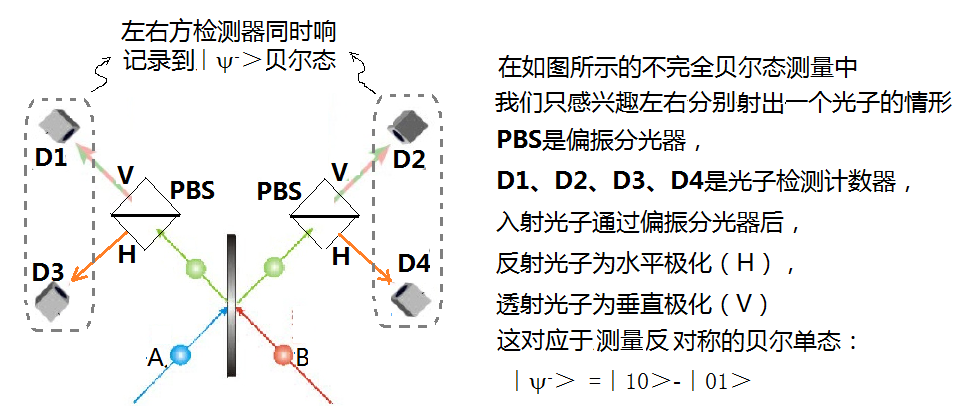
如上图所示,在光子离开分光器(BS)出来的两个途径上各置一个偏振分光器(PBS)。光子经过PBS后,按概率分成垂直偏振和水平偏振两条路。左右两边的光子的两种可能性分别由侦测器D1、D3和D2、D4探测。我们仅仅当左右方两个检测器同时侦测到光子时,才作记录,这就是所谓的同时符合测量(coincidence measurement)。这样的设置意谓每一出来的途径必须有一个光子,所以只有两个可能:两个光子经过分光器时都继续前进或同时被反射,因为这两种情形是无法区分的,所以出来后之两光子态是这两种情形之状态之线性叠加,其振幅大小相同而符号相反。因此,在符合测量设置下,只有贝尔单态|y->是容许的。这时,我们测量到了贝尔单态,而原来两光子之状态也崩溃成贝尔单态。
1997年,塞林格所领导的奥地利国际研究小组第一次在实验上实现了量子隐形传输。2004年,这个小组又利用多瑙河底的光纤信道,成功地将量子态隐形传输距离提高到600米。之后,中国科大——清华联合小组在北京八达岭与河北怀来之间,架设长达16公里的自由空间量子信道,并取得了一系列关键技术突破,最终在2009年成功实现了世界上迄今为止最远距离的量子态隐形传输。
量子隐形传态实验过程的简化原理图如下所示:
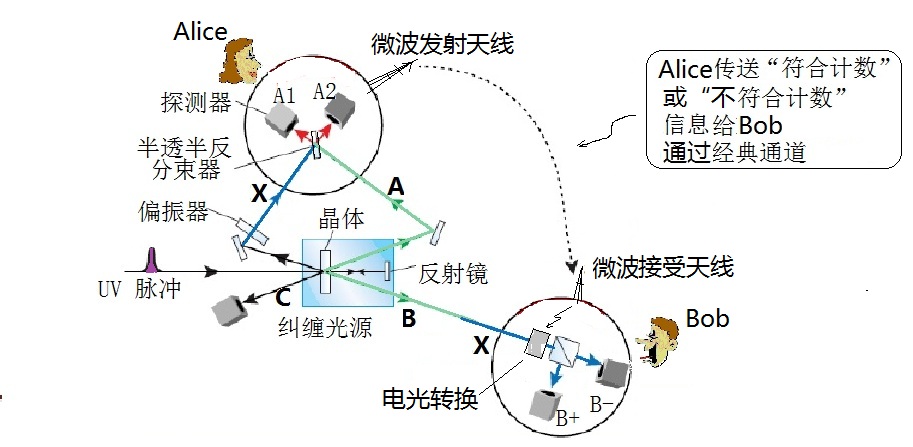
图中心纠缠光源发出的孪生光子A和B分别传送给Alice和Bob。 Alice 处有半透半反分光器BS等,对A以及准备隐形传态的光子X,作刚才我们所描述的“同时符合”贝尔测量,将测量的结果:“符合”或“不符合”,通过经典通道,比如微波天线,发射给远在另一端(多瑙河对岸)的Bob。然后,Bob便需要对他所拥有的B,或者说是,从多瑙河底的光纤信道(量子通道)传过来的光子,作一些我们上一节中提到过的“变换处理”。
比较起Alice的“贝尔测量”来说,Bob的“变换处理”操作要简单多了,因为实际上,在Alice用X和A完成贝尔测量的那一霎那,X、A、B三粒子之间,已经完成了“纠缠转移”:原来不纠缠的X和A纠缠起来,光子X原来量子态的大部分信息,已经转移到B。比如在Alice作的“同时符合”贝尔测量情况下,Bob只需要根据从微波天线接受到的信息,对光纤信道传来的光子,作点小变换:如果微波信息是“符合”,什么也不作;如果微波信息是不“符合”,则将传来的光子的偏振方向变成与原方向垂直。上面所说的目的,用得到的微波信息,连到一个电光转换开关,再控制偏振器,即可达到。像在上图中,Bob的圆圈中所显示的那样。
到此为止,原来的光子X的所有信息都转移到了Bob所拥有的光子B上。而实际上,Alice和Bob从始至终都对X上的这些信息一无所知,他们唯一所知道的只是: 最后,X和A成为纠缠单态,Bob的粒子有了原来X的所有性质,隐形传态完成了。
在量子隐形传态的实验中,调节每个光子之间的时间差,做到两个光子必须“同时”到达测量仪器,对隐形传态的成功与否至关重要。
“贝尔测量”也是影响传态保真度的重要因素。因为利用线性光学元件,不能完全区分四个贝尔态。因此,要实现完全的贝尔测量,就需要采取另外一些办法。一个方法是使用非线性的光学器件(见参考资料)。
另外一条路就是采取“连续变量”纠缠源来实现量子隐形传态。
我们在此文中所叙述的量子纠缠及其在量子信息中的应用,基本是基于以单光子偏振态为代表的 “分离变量”方法。实际上,也有不少实验室研究所谓“连续变量”的量子信息技术。连续变量量子信息,是以光场正交振幅和正交位相分量为代表。“分离变量”对应于有限维的状态空间,可以用简单的量子力学算符和方程准确描述,而“连续变量”对应于无限维的状态空间,解释起来不容易。两种方式各有优点与不足,比如对量子隐形传态来说,用连续变量方法,可以做到完全的贝尔测量,理想情况下的贝尔探测效率可达100%。在此我们不多谈两种方式的优缺点,目前也有人提出hybrid 的方案,即是将分离变量和连续变量量子资源结合起来,发展混合型的量子信息技术。
可喜的是,对量子信息的研究和实验方面,中国的学者们,走在了国际科研的前沿。除了使用分离变量方法的中科大-清华团队之外,山西大学光电研究所在连续变量量子信息方面做了很多突出的工作,他们的实验室,不仅在国内连续变量领域是独此一家,在世界上也可算是这方面几个有代表性的实验室之一。他们在2004年,最早实现了连续变量的量子隐形传输。2006年,他们的研究团队利用连续变量量子纠缠,设计和实现了量子保密通信,并证明了它在长距离传输中的安全性(见参考资料)。
参考资料:
第一次量子隐形传态:D. Bouwmeester, J. W. Pan, K. Mattle, M. Eibl, H.Weinfurter, and A.Zeilinger, "Experimental quantum teleportation,"Nature 390 (6660),575-579 (1997).
中科大和清华团队文章:Xian-Min Jin, Ji-Gang Ren, Bin Yang, Zhen-Huan Yi,Fei Zhou, Xiao-Fan Xu, Shao-Kai Wang, Dong Yang, Yuan-Feng Hu, Shuo Jiang, TaoYang, Hao Yin, Kai Chen, Cheng-Zhi Peng and Jian-Wei Pan. ExperimentalFree-Space Quantum Teleportation,Nature Photonics, 4, 376-381(2010).
连续变量:“Experimental procedures for entanglementverification”, on Phys. Rev. A 75 , 052318 (2007);
混合型量子信息:“Optical hybrid approaches to quantum information” on Laser & Photonics Reviews , February 25 , 2010。
连续变量量子隐形传输:Phys.Rev.Lett.93.250503;
连续变量量子保密通信:Europhys. Lett. 87, 20005 (2009); Phys. Rev. A 74,062305 (2006)。
(全文完)
No comments:
Post a Comment
光子具有自旋角动量和轨道角动量,如果你看不懂这两个词,没关系,只要明白它们是两个自由度就够了。在以前的实验中,传的只是轨道角动量的状态。但是如果你想真正传输一个光子的完整状态,应该把这两个自由度的状态都传过去。潘建伟
科普量子瞬间传输技术,包你懂!
作者:@中科大胡不归
我的专业是理论物理化学,按说没资格科普量子信息。不过我好歹懂得比公众多一些,并且请教了一位潘建伟院士组里的同事陈博士。虽然陈博士不是这篇文章的作者,而且一再声称他做的不是这一块,对整个量子信息也了解有限(这是科研工作者的标准态度,有一分证据说一分话),但还是提供了很多深入浅出的解读,特此鸣谢。于是乎,我觉得我对这项工作有一定的宏观了解,可以向公众解释解释了。虽然在内行看来很粗浅,但至少可以澄清一些误解,让你明白这项成果实际上是什么,不是什么,在科学史上处于什么位置,重要性有多高。我的叙述会力求简明,让高中以上文化水平的人都能看明白,同时力求准确,给出正确的科学图像。其实准确的表述往往比似是而非的表述更容易理解,这是看了很多半通不通的报道和教材之后的感受。总之,包你懂!如果还是不懂……再看一遍!:-)
这项工作是2月26日以封面标题的形式发表在国际顶级科学期刊《自然》(Nature)上的,文章标题是《单个光子的多个自由度的量子隐形传态》(“Quantum teleportation of multiple degrees of freedom of a single photon”)。这里新的成果是“多个自由度”,因为1997年就实现了单个光子的单个自由度的量子隐形传态。那么,什么是光子?(这个问题大家应该都知道,光子是光的最小单元,日常见到的一束光中包含非常多个光子。)什么是自由度?什么是量子?什么是态?什么是量子传态?
一个物理量如果存在最小的不可分割的基本单位,我们就说这个物理量是量子化的,把这个最小单位称为量子。光子就是光量子,一束光至少包含一个光子,再少就不存在了。实验发现,原子中电子的能量不是连续变化的,而是只能取一些分立的值,也就是说,原子中的电子能量是量子化的。量子化是微观世界的普遍现象。20世纪上半叶(主要是从1900年到1930年),普朗克、爱因斯坦、德布罗意、玻尔、海森堡、薛定谔、狄拉克、玻恩、泡利等伟大的物理学家们创立了量子力学,这是我们目前对微观世界最准确的描述。相对论几乎是爱因斯坦独力创造出来的,量子力学却是群星璀璨的产物。爱因斯坦在其中也发挥了非常重要的作用(提出光量子,这是他得诺贝尔物理学奖的原因,居然不是相对论!),但并不是最重要的,最重要的两个贡献者是普朗克和海森堡。不过上面无论哪一位,都比在世的物理学家伟大多了(杨振宁可能跟泡利相差不是很远?),这是时代的垂青,个人无法改变的。
量子力学描述世界的语言跟经典力学有根本区别。经典力学描述一个粒子的状态,说的是它在什么位置,具有什么动量。不言而喻的是,在任何一个时刻这个粒子总是位于某个位置,具有某个动量,即使你不知道是多少。量子力学描述一个粒子的状态,却是给出一个态函数或者称为态矢量,这个态矢量不是位于日常所见的三维空间,而是位于一个数学抽象的线性空间。在这里我们不需要深究这是个什么空间,关键在于两个态矢量之间可以进行“内积”的运算。内积是什么?在三维空间中,两个长度为1的单位矢量a和b做内积(a, b),得到的是它们夹角的余弦,即两个矢量方向相同时得到1,方向相反时得到-1,互相垂直时得到0,所以内积也可以理解为一个矢量在另一个矢量上的投影。对两个态矢量也可以求这样的内积,结果是个复数(即有实部虚部,不一定是实数),而这个复数的绝对值小于等于1。
好,现在不可思议的新概念来了:对于任何一个物理量P(例如位置、动量),态矢量都可以分为两类,一类具有确定的P,称为P的本征态,P的取值称为这个本征态的本征值;另一类不具有确定的P,称为P的非本征态。非本征态比本征态多得多,如同无理数比有理数多得多。也就是说,绝大多数情况下,一个粒子是没有确定的位置的!等等,什么叫做“没有确定的位置”?是因为粒子跑得太快了,我们看不清吗?量子力学说的不是这种常规(而错误)的理解,而是说:非本征态是一个客观真实的状态,跟本征态同样客观真实,它没有确定的位置是因为它本质上就是如此,而不是因为我们的信息不全。来打个比方,有些状态可以用指向上下左右的箭头来表示,于是你定义“方向”为一个物理量,但是还有些状态是一个圆!圆状态跟箭头状态同样真实,只是没有确定的方向而已。
但是读者还会困惑,因为我们总是可以用仪器去测量粒子的位置,测量的结果总是粒子出现在某个地方,而不是同时出现在两个地方,或者哪里都测量不到。好,下面就是量子力学的关键思想:对P的本征态测量P,粒子的状态不变,测得的是这个本征态的本征值。而对P的非本征态s测量P,会使粒子的状态从s变成某个P的本征态f,概率是s与f的内积的绝对值的平方|(s, f)|^2,发生这个变化后测得的就是f的本征值。用上面的例子来说,对箭头状态测方向,状态不变,得到的就是箭头的方向;对圆状态测方向,圆状态会以相同的几率变成任何一个箭头状态,得到的是这个新的箭头状态的方向。对位置的非本征态测量位置,就会测得粒子出现在某个随机的位置,而出现在空间所有位置的几率之和等于1。怎么知道测量结果是随机的呢?制备多个具有相同状态的粒子,把实验重复多次,就会发现实验结果每次都不一样。没错,量子力学具有本质的随机性,同样的原因可以导致不同的结果,这是跟经典力学的又一大区别。
你也许会觉得上面这些说法简直莫名其妙,但是现在绝大多数科学家都对它们奉若圭臬。为什么呢?因为这套奇怪的理论跟实验符合得很好,而经典力学却不能。当然,这是哲学性的原因,而操作性的原因很简单:现在的科学家受的都是量子力学的教育。普朗克有一句非常有趣的话:“新的科学真理并不是由于说服它的对手取得胜利的,而是由于它的对手死光了,新的一代熟悉它的人成长起来了。”诚哉斯言!
事实上,现在仍然有不少人对量子力学提出各种各样的挑战,包括不少专业科学家,民科就更多了(当然挑战相对论的民科更多)。历史上,挑战量子力学的势力更加强大,其中的带头大哥就是--爱因斯坦!老爱坚信粒子应该具有确定的位置和动量,世界的演化应该是决定性的,对前面说的量子力学的不确定性和随机性十分不满。用他自己的话来说,他相信“没有人看月亮的时候,月亮仍然存在”,以及“上帝不掷骰子”。
如果是一般人,表达完信念也就没事了。但爱因斯坦是超级伟大的科学家,神一样的人物,他不会满足于只做口舌之争,而是要设计一个判决性的实验,以可验证的方式证明量子力学的错误。于是乎,1935年,爱因斯坦(Einstein)、波多尔斯基(Podolsky)和罗森(Rosen)提出了一个思想实验,后人用他们的首字母称为EPR实验。你可以制备两个粒子A和B的“圆”态,使得在这个状态中两个粒子的某个性质(如电子的自旋角动量、光子的偏振)相加等于零,而单个粒子的这个性质不确定。这样一对粒子称为EPR对。然后你把这两个粒子在空间上分开很远,任意的远,然后测量粒子A的这个性质。好比你测得A是“上”,那么你就立刻知道了B现在是“下”。问题是,既然A和B已经离得非常远了,B是怎么知道A发生了变化,然后发生相应的变化的?EPR认为A和B之间出现了“鬼魅般的超距作用”,信息传递的速度超过光速,违反相对论。所以,量子力学肯定有错误。
这个问题非常深邃,直到现在都不断给人以启发。不过量子力学的正统卫道士有一个标准回答:处于“圆”态的A和B是一个整体,当你对A进行测量的时候,A和B是同时发生变化的,并不是A变了之后传一个信息给B,B再变化,所以这里没有信息的传递,不违反相对论。这个回答怎么样?无论你信不信,反正我信了。不过爱因斯坦一直都不信,以这个他参与创建的理论的反对者的身份走完了一生。
在爱因斯坦的时代,EPR实验只能在头脑中进行。随着科技的进步,这个实验可以实现了。1980年代,阿斯佩克特等人做了EPR实验,结果你猜怎么着?完全跟量子力学的预言符合!真的是你测得一个EPR对中的A是“上”的时候,B就变成了“下”。本来是设计出来否定量子力学的,反而验证了量子力学的正确性。这种事在科学史上屡见不鲜。17世纪的时候,牛顿主张光是粒子,惠更斯主张光是波动。牛顿按照惠更斯的理论计算出一个现象:把一束光射向一个不透明的小圆片,在圆片的背后中心位置会出现一个亮点,而不是暗点。牛顿认为这是不可能的,宣布驳倒了惠更斯。可是别人一做这个实验,发现真的就是如此,结果成了牛顿亲手证明惠更斯的正确。这正应了尼采的话:“杀不死我的,使我更强大!”
EPR现象既然是一个真实的效应,而不是爱因斯坦等人以为的悖论,人们就想到利用它。量子隐形传态(quantum teleportation)就是一个重要的应用。英文单词teleportation就是科幻艺术中biu的一声把人传过去的瞬间传输,tele是远,port是传,所以小编们报道这种新闻总是配传人的图片,《星际迷航》中的Spock发来贺电!可是,在量子信息研究中实际做的是把一个粒子A的量子态传输给远处的另一个粒子B,让B复制A的状态,注意传的是状态而不是粒子。当然你可以说传人也是把人的所有原子的状态传到远处的另外一堆原子上,组合成一个同样的人。OK我没意见,只不过为了避免混淆,中国的科学家们还是小心谨慎地把teleportation翻译成了隐形传态。
量子隐形传态是怎么操作的呢?基本思路是这样:让第三个粒子C跟B组成EPR对,而C跟A离得很近,跟B离得很远。让A按照某个密码跟C发生相互作用,改变C的状态,于是B的状态也发生了相应的变化。再通过经典的通讯手段(比如电话、光缆)把密码告诉B那边的人,对B按照密码进行反向操作,就得到了A的状态。这里的基本元素包括作为中介的C、密码和传输密码的经典信道。
量子隐形传态是在什么时候实现的?答案是1997年,当时潘建伟在奥地利维也纳大学的塞林格(Zeilinger)教授组里读博士,他们在《自然》上发表了一篇题为《实验量子隐形传态》(“Experimental quantum teleportation”)的文章,潘建伟是第二作者。这篇文章后来入选了《自然》杂志的“百年物理学21篇经典论文”,跟它并列的包括伦琴发现X射线、爱因斯坦建立相对论、沃森和克里克发现DNA双螺旋结构等等,这个阵容强大得吓死人。当然,量子隐形传态的重要性不能和那些神级成果相提并论,不过也已经相当了不起了,尤其是在基础科学已经很久没有革命的当代。
现在终于可以说到潘建伟研究组最新的这个工作了。1997年实现的是单个光子的单个自由度的量子隐形传态,现在实现的是单个光子的多个自由度的量子隐形传态。自由度是什么?自由度就是描述一个体系所需的变量的数目。例如一个静止在一条线上的粒子,描述它只需要一个数,自由度就是1。静止在一个面上的粒子,自由度就是2。三维空间中的静止粒子,自由度就是3。描述三维空间中一个运动的粒子,需要知道位置的3个分量和动量的3个分离,自由度是6。光子具有自旋角动量和轨道角动量,如果你看不懂这两个词,没关系,只要明白它们是两个自由度就够了。在以前的实验中,传的只是轨道角动量的状态。但是如果你想真正传输一个光子的完整状态,应该把这两个自由度的状态都传过去。潘建伟研究组实现的就是这件事。所以完整意义的量子隐形传态,应该说是2015年才实现的。
这两个实验之间为什么隔了18年之久呢?因为前面说的全都是理论,而在实验操作中有非常多的技术困难。为了解决这些困难,他们“巧妙地设计了利用单光子非破坏测量技术实现自旋和轨道角动量多自由度贝尔态测量的新方案,制备了国际上最高亮度的自旋-轨道角动量超纠缠源、高效率的轨道角动量测量器件,搭建了6光子11量子比特的自旋-轨道角动量纠缠实验平台”。不要问我这些是什么意思,对量子信息的业外人士来说这些是技术细节了。重要的是,这些技术进步都非常新颖,非常困难,通过这些实验手段的创新,他们终于达到了多自由度隐形传态的目的。其实这个目的实现得还不是非常完美。文章摘要里有一句“传输的保真度(fidelity)位于0.57至0.68之间”,也就是说,有40%左右的可能性传输失败!这是实验中的种种噪声、损耗造成的,不是理论的限制,以后可以继续提高。在单自由度的传态中,保真度已经能达到99%以上了。这是现代科研的常态,在一个看似简单的故事下面隐含着无数的技术细节。这是隔行如隔山的来源,也是民科在当代的作用远远比历史上小的原因。
知道了这项成果是什么,我们可以来回答它不是什么了。很遗憾,它不是biu的一声把人传走。当然,可以说是朝这个方向前进了一步,而且是一大步。多大的一步?如果用《老子》的话:“道生一,一生二,二生三,三生万物。”1997年是实现了道生一,这次是实现了一生二。不过,离传人有多远的距离呢?可以这样估算。12克碳原子是1摩尔,即6*10^23个。人的体重如果是60公斤,就大约有5000摩尔的原子,即3*10^27个。描述一个原子的状态,我不知道要多少个自由度,姑且算作10个吧。那么要描述一个人,就需要10^28量级的自由度。我们现在刚刚从1进步到了2……就连这个2也不是非常稳定的,还有40%的几率传错,想想如果你身上1%的细胞出了错会怎么样?所以,嗯,我们的征途是星辰大海!骚年,向着夕阳奔跑吧!
由于这项工作的重要性,《自然》在同一期上评论道:“该实验为理解和展示量子物理的一个最深远和最令人费解的预言迈出了重要的一步,并可以作为未来量子网络的一个强大的基本单元。”这是一个恰如其分的评价。如果你要问,能不能得诺贝尔奖?我不好说,不过如果真有一天得奖,塞林格应该在前面,因为道生一肯定比一生二重要。当然,潘建伟和他的团队都还很年轻,他们有无限的可能性,将来因为其它的成就得诺贝尔奖也未可知。科学最大的魅力之一,就是一切皆有可能。(来,干了这碗鸡汤!)
潘建伟研究组是量子信息的世界领导者之一,当然不好说是最先进的,因为在欧洲、美国也有跟他们差不多水平、各有千秋的研究组。在量子保密通信这个领域他们做得尤其突出,创造了多项传输距离的世界纪录,已经有多个政务网投入实用,并将在2016年发射量子卫星。目前中国很少有领域具有这样领先的世界地位,弥足珍贵。
值得特别强调的是,中国的量子信息绝不是一花独放,而是百花争春。仅仅在科大,比较大的研究组就有潘建伟院士、郭光灿院士、杜江峰教授三家,比较小的我就数不过来了。顺便说一下,最近杜江峰研究组在3月6日的《科学》杂志上发表了题为《日常环境下单蛋白质的自旋共振谱》(“Single-protein spin resonance spectroscopy under ambient conditions”)的文章,周荣斌研究组在1月15日的《细胞》(Cell)杂志上发表了题为“Dopamine controls systemic inflammation through inhibition of NLRP3 inflammasome”的文章。在2015年的前3个月,科大在《自然》、《科学》和《细胞》这三大国际顶级期刊(合称CNS)上各发了一篇,堪称开门红。当然,对CNS大惊小怪仍然是中国的大学科研水平有限的证据,因为还是少嘛。什么时候CNS多得大家都不当回事了,不再关心文章发在什么地方,只专注成果的科学重要性,那就真的是世界一流大学了。
据我了解,中国的量子信息研究是从1990年代开始的。那时郭光灿从量子光学转向量子信息,迎来了事业的高峰。潘建伟那时只是研究生,现在已经是国际领军人物了。从这些轨迹可以看出,一个国家的科学可以进步得有多快。1999年左右,杨振宁到科大演讲《近代科学进入中国的回顾与前瞻》,结论是:“以下的几个长远的因素是使得一个社会、一个国家能够有辉煌的科技发展的必要条件。第一个是需要有聪明的年轻人,有头脑做科学研究;第二是需要有重视纪律、重视忍耐心、重视勤奋的社会传统;第三要有决心;第四要有经济条件。……中国在20世纪里有前三者,到了21世纪我认为将四者具备,所以我对21世纪中国科技的发展是绝对乐观的。”当时我十分不以为然,因为中国有太多的问题,腐败,专制,贫富差距,世风日下……所以,杨先生,您是不是老糊涂了?不错,当时我倾向于崩溃论,对中国的前途十分悲观。后来随着眼界的扩大,越来越发现杨振宁讲的是完全正确的。虽然他这些道理看起来无比的质朴,简直是土得掉渣,但实际上是“重剑无锋,大巧不工”。科学大师关于科学发展的眼光确实比我们高得多,不服不行。你说中国没人才,耐心培养不就是了?以中国人的天分,说不定一搞就搞出个国际领导者来。科学最大的魅力之一,就是一切皆有可能。(来,再干了这碗鸡汤!)
在这里我要讲一个故事。美国物理学家拉比(1898-1988)年轻的时候去欧洲留学,发现美国的物理杂志《物理评论》是被一年一次用船运过去的,说明在欧洲科学界看来美国的物理学根本不值得重视。拉比暗下决心振兴美国物理学,回国后担任了《物理评论》的主编,如今这本杂志是世界物理学界最著名的期刊之一,美国的物理学是世界上最先进的。美国化学家鲍林(1901-1994)也是在去欧洲留学之后,把美国的化学提升到了世界最先进水平。
如果当时有“冷静党”跳出来说美国人不行,永远赶不上欧洲,也能找到无数的证据。而这样的“冷静党”在中国一抓一大把,正如@吏部尚书吉哈克 所说:“中国人的意识已经跟不上中国的发展了。国家顶尖的科研人员已经搞的是人类科学中顶尖的那些东西了,而民众甚至人大委员却还在迷恋日本的马桶圈和电饭煲……脚用30年走了人家300年的路,脑子却留在了30年前……”
你愿意向顶尖的科研人员看齐吗?中国最大的魅力之一,就是一切皆有可能。
Two quantum properties teleported together for first time
Twice the fun: teleporting two properties of a photon
Alice and Bob
Quantum teleportation first appeared in the early 1990s after four researchers, including Charles Bennett of IBM in New York, developed a basic quantum teleportation protocol. To successfully teleport a quantum state, you must make a precise initial measurement of a system, transmit the measurement information to a receiving destination and then reconstruct a perfect copy of the original state. The "no-cloning" theorem of quantum mechanics dictates that it is impossible to make a perfect copy of a quantum particle. But researchers found a way around this via teleportation, which allows a flawless copy of a property of a particle to be made. This occurs thanks to what is ultimately a complete transfer (rather than an actual copy) of the property onto another particle such that the first particle loses all of the properties that are teleported.The protocol has an observer, Alice, send information about an unknown quantum state (or property) to another observer, Bob, via the exchange of classical information. Both Alice and Bob are first given one half of an additional pair of entangled particles that act as the "quantum channel" via which the teleportation will ultimately take place. Alice would then interact the unknown quantum state with her half of the entangled particle, measure the combined quantum state and send the result through a classical channel to Bob. The act of the measurement itself alters the state of Bob's half of the entangled pair and this, combined with the result of Alice's measurement, allows Bob to reconstruct the unknown quantum state. The first experimentation teleportation of the spin (or polarization) of a photon took place in 1997. Since then, the states of atomic spins, coherent light fields, nuclear spins and trapped ions have all been teleported.
But any quantum particle has more than one given state or property – they possess various "degrees of freedom", many of which are related. Even the simple photon has various properties such as frequency, momentum, spin and orbital angular momentum (OAM), which are inherently linked.
More than one
Teleporting more than one state simultaneously is essential to fully describe a quantum particle and achieving this would be a tentative step towards teleporting something larger than a quantum particle, which could be very useful in the exchange of quantum information. Now, Chaoyang Lu and Jian-Wei Pan, along with colleagues at the University of Science and Technology of China in Hefei, have taken the first step in simultaneously teleporting multiple properties of a single photon.In the experiment, the team teleports the composite quantum states of a single photon encoded in both its spin and OAM. To transfer the two properties requires not only an extra entangled set of particles (the quantum channel), but a "hyper-entangled" set – where the two particles are simultaneously entangled in both their spin and their OAM. The researchers shine a strong ultraviolet pulsed laser on three nonlinear crystals to generate three entangled pairs of photons – one pair is hyper-entangled and is used as the "quantum channel", a second entangled pair is used to carry out an intermediate "non-destructive" measurement, while the third pair is used to prepare the two-property state of a single photon that will eventually be teleported.
Tricky protocol: comparative measurements and teleportation
On the other hand, if the comparative measurement showed that A's polarization as compared with B differed by 90° (i.e. A and B are orthogonally polarized), then we would rotate C's field by 90° with respect to that of A to make a perfect transfer once more. Simply put, making two comparative measurements, followed by a well-defined rotation of the still-unknown polarization or OAM, would allow us to teleport A's properties to C.
Perfect protocol
One of the most challenging steps for the researchers was to link together the two comparative measurements. Referring to the "joint measurements" box in the image above, we begin with the comparative measurement of A and B's polarization (CM-P). From here, either one of three scenarios can take place – one photon travels along path 1 to the middle box (labelled "non-destructive photon-number measurement"); no photons enter the middle box along path 1; or two single photons enter the middle box along path 1.The middle box itself contains the second set of entangled photons mentioned previously (not shown in figure) and one of these two entangled photons is jointly measured with the incoming photons from path 1. But the researcher's condition is that if either no photons or two photons enter the middle box via path 1, then the measurement would fail. Indeed, what the middle box ultimately shows is that exactly one photon existed in path 1, and so exactly one photon existed in path 2, given that two photons (A and B) entered CM-P. To show that indeed one photon existed in path two required the third and final set of entangled photons in the CP-OAM box (not shown), where the OAM's of A and B undergo a comparative measurement.
The measurements ultimately result in the transfer or teleportation of A's properties onto C – although it may require rotating C's (as yet unknown) polarization and OAM depending on the outcomes of the comparative measurements, but the researchers did not actually implement the rotations in their current experiment. The team's work has been published in the journal Nature this week. Pan tells physicsworld.com that the team verified that "the teleportation works for both spin-orbit product state and hybrid entangled state, achieving an overall fidelity that well exceeds the classical limit". He says that these "methods can, in principle, be generalized to more [properties], for instance, involving the photon's momentum, time and frequency".
Verification verdicts
Physicist Wolfgang Tittel from the University of Calgary, who was not involved in the current work (but wrote an accompanying "News and Views" article in Nature) explains that the team verified that the teleportation had indeed occurred by measuring the properties of C after the teleportation. "Of course, the no-cloning theorem does not allow them to do this perfectly. But it is possible to repeat the teleportation of the properties of photon A, prepared every time in the same way, many times. Making measurements on photon C (one per repetition) allows reconstructing its properties." He points out that although the rotations were not ultimately implemented by the researchers, they found that "the properties of C differed from those of A almost exactly by the amount predicted by the outcomes of the comparative measurements. They repeated this large number of measurements for different preparations of A, always finding the properties of C close to those expected. This suffices to claim quantum teleportation".While it is technically possible to extend Pan's method to teleport more than two properties simultaneously, this is increasingly difficult because the probability of a successful comparative measurement decreases with each added property. "I think with the scheme demonstrated by [the researchers], the limit is three properties. But this does not mean that other approaches, either other schemes based on photons, or approaches using other particles (e.g. trapped ions), can't do better," says Tittel.
Pan says that to teleport three properties, their scheme "needs the experimental ability to control 10 photons. So far, our record is eight photon entanglement. We are currently working on two parallel lines to get more photon entanglement." Indeed, he says that the team's next goal is to experimentally create "the largest hyper-entangled state so far: a six-photon 18-qubit Schrödinger cat state, entangled in three degrees-of-freedom, polarization, orbital angular momentum, and spatial mode. To do this would provide us with an advanced platform for quantum communication and computation protocols".
The work is published in Nature.